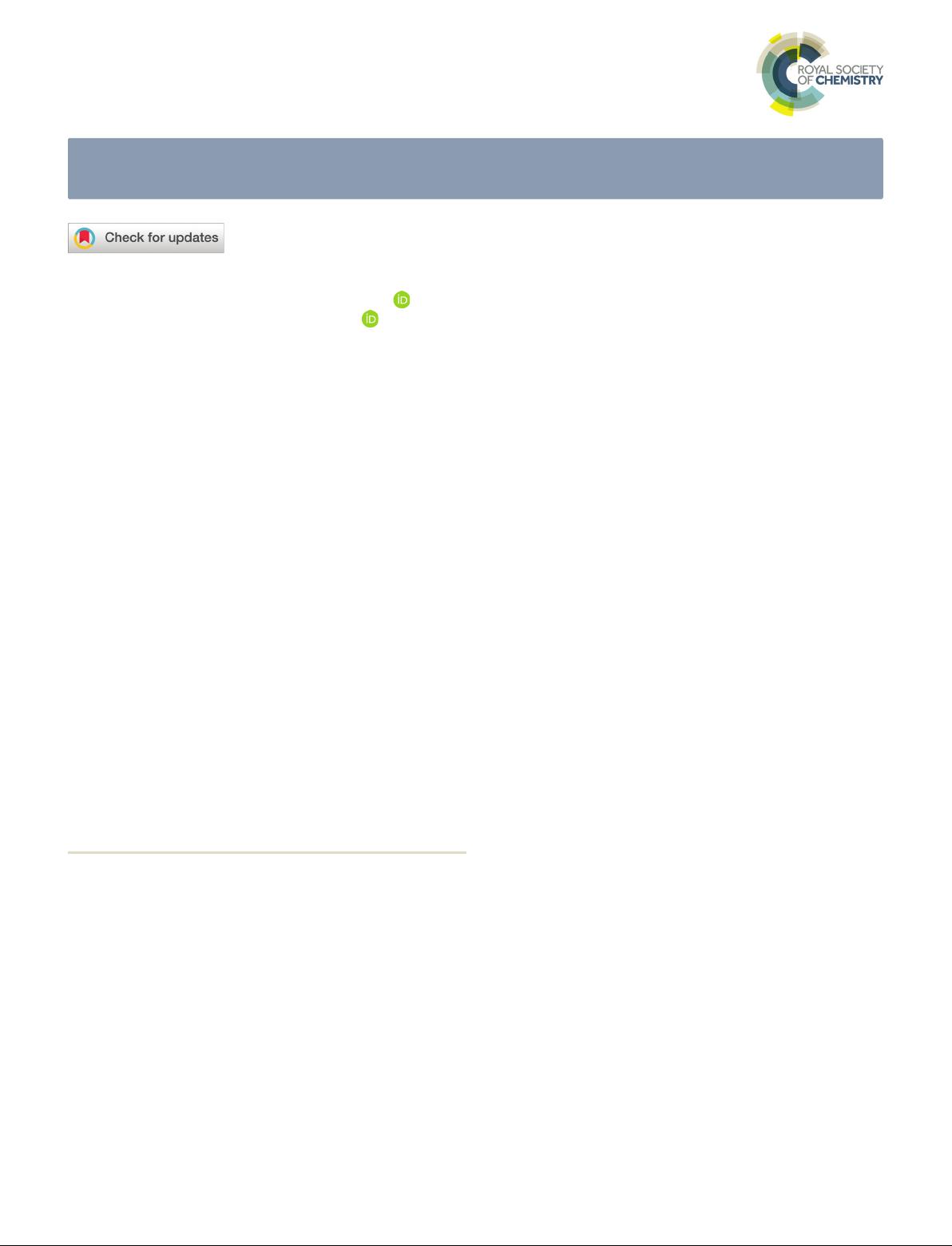
Nanoscale
PAPER
Cite this: Nanoscale, 2019, 11, 1762
Received 28th September 2018,
Accepted 19th December 2018
DOI: 10.1039/c8nr07894g
rsc.li/nanoscale
2D group-VA fluorinated antimonene: synthesis
and saturable absorption†
Guangju Zhang, ‡
a
Xian Tang,‡
b
Xing Fu,
a
Weicheng Chen,
c
Babar Shabbir,
d
Han Zhang, *
b
Qiang Liu*
a
and Mali Gong*
a
A new derivative of antimonene named fluorinated antimonene was synthesized using the method of
ionic liquid-assisted electrochemical exfoliation and synchronous fluorination. Passive Q-switched pulses
were produced from a Nd:LuAG laser with fluorinated antimonene, having a pulse width of 326.7 ns and a
repetition rate of 733.1 kHz demonstrating its potential application as a saturable absorber. Density func-
tional theory calculations revealed that compared with pure antimonene with an indirect bandgap, fluori-
nated antimonene exhibits a direct bandgap modulated by the fluorination degree showing that fluori-
nated antimonene would be applied as optical devices beyond its application as a saturable absorber.
Introduction
Since the discovery of graphene, 2D materials have attracted
extensive attention in physics, chemistry and materials science
due to their unusual and outstanding properties including
high mobility,
1
excellent mechanical performance,
2
and so
forth. Beyond the scientific research revealing these properties,
great potential applications of 2D materials, such as electronic
and optoelectronic devices,
3–5
biosystems,
6
and thermal man-
agement,
7
motivate scientists to explore new families of 2D
materials. 2D group-VA materials are the most recently discov-
ered which have expanded the 2D material community besides
graphene, topological insulators and transition-metal dichal-
cogenides (TMDs).
8
2D group-VA materials possess a 3s
2
3p
3
outmost shell, and the existence of the sp
3
-hybridized states
depends on the monolayer structures. In the buckled honey-
comb monolayer, sp
3
-hybridizations may be partially formed
between in-plane sp
2
and out-of-plane p
z
orbitals. If the mono-
layer has a flat honeycomb structure, no sp
3
-hybridizations will
exist.
9
This endows 2D group-VA materials with some unique
properties and potential applications compared to other 2D
materials. For example, their electronic bandgap varying
between 0.36 and 2.62 eV can be determined by the thickness,
which would inspire great potential for applications emphasiz-
ing a broadband photoresponse.
Saturable absorption of light widely exists in 2D
materials.
10–26
Nowadays, considering the importance of
pulsed lasers in science and technology, this property endows
2D materials with extremely high values in related research
studies. As TMDs normally possess a moderate bandgap (1 eV
for bulk and 2 eV for the monolayer
27,28
) compared to 2D
group-VA materials, in theory, 2D group-VA materials have
saturable absorption in a wider spectral region compared to
TMDs, which has been demonstrated by phosphorene, the
firstly discovered 2D group-VA material.
29
Recent research
studies have determined phosphorene to have excellent satur-
able absorption spanning from 0.4 to 3 μm by adopting it as a
saturable absorber (SA) in pulsed lasers.
30–33
On the other
hand, as the firstly and most intensively studied SA in 2D
materials, graphene possesses a relatively weak absorption in
the monolayer than phosphorene.
27,34,35
However, the intrinsic
instability of phosphorene has been an essential obstacle hin-
dering its long-term use.
36
Most recently, the 2D group-VA
family has been expanded to include arsenene,
9,37
antimonene
8,38–43
and bismuthene,
10,44–46
and antimonene
has been found to possess robust ambient stability.
47
In pre-
vious research studies,
11,39
few-layer antimonene was revealed
to be beneficial for developing antimonene-based and broad-
band-photoresponse devices in passive Q-switched (QS) solid-
† Electronic supplementary information (ESI) available: Snapshots of the syn-
thesis process, large-area AFM image, electronic structures, and photo-
luminescence spectrum of fluorinated antimonene. See DOI: 10.1039/
c8nr07894g
‡ These authors contribute equally to this work.
a
Center for Photonics and Electronics, Department of Precision Instruments,
Tsinghua University, Beijing 100084, China. E-mail: qiangliu@mail.tsinghua.edu.cn,
gongml@mail.tsinghua.edu.cn
b
Shenzhen Engineering Laboratory of Phosphorene and Optoelectronics,
Collaborative Innovation Center for Optoelectronics Science and Technology, and Key
Laboratory of Optoelectronics Devices and Systems of Ministry of Education and
Guangdong Province, Shenzhen University, Shenzhen 518060, China.
E-mail: hzhang@szu.edu.cn
c
School of Physics and Optoelectronic Engineering, Foshan University, Foshan
528000, China
d
Department of Materials Science and Engineering, and ARC Centre of Excellence in
Future Low-Energy Electronics Technologies (FLEET), Monash University, Clayton,
VIC 3800, Australia
1762 | Nanoscale,2019,11,1762–1769 This journal is © The Royal Society of Chemistry 2019
Published on 20 December 2018. Downloaded by Shenzhen University on 3/6/2019 2:03:03 AM.