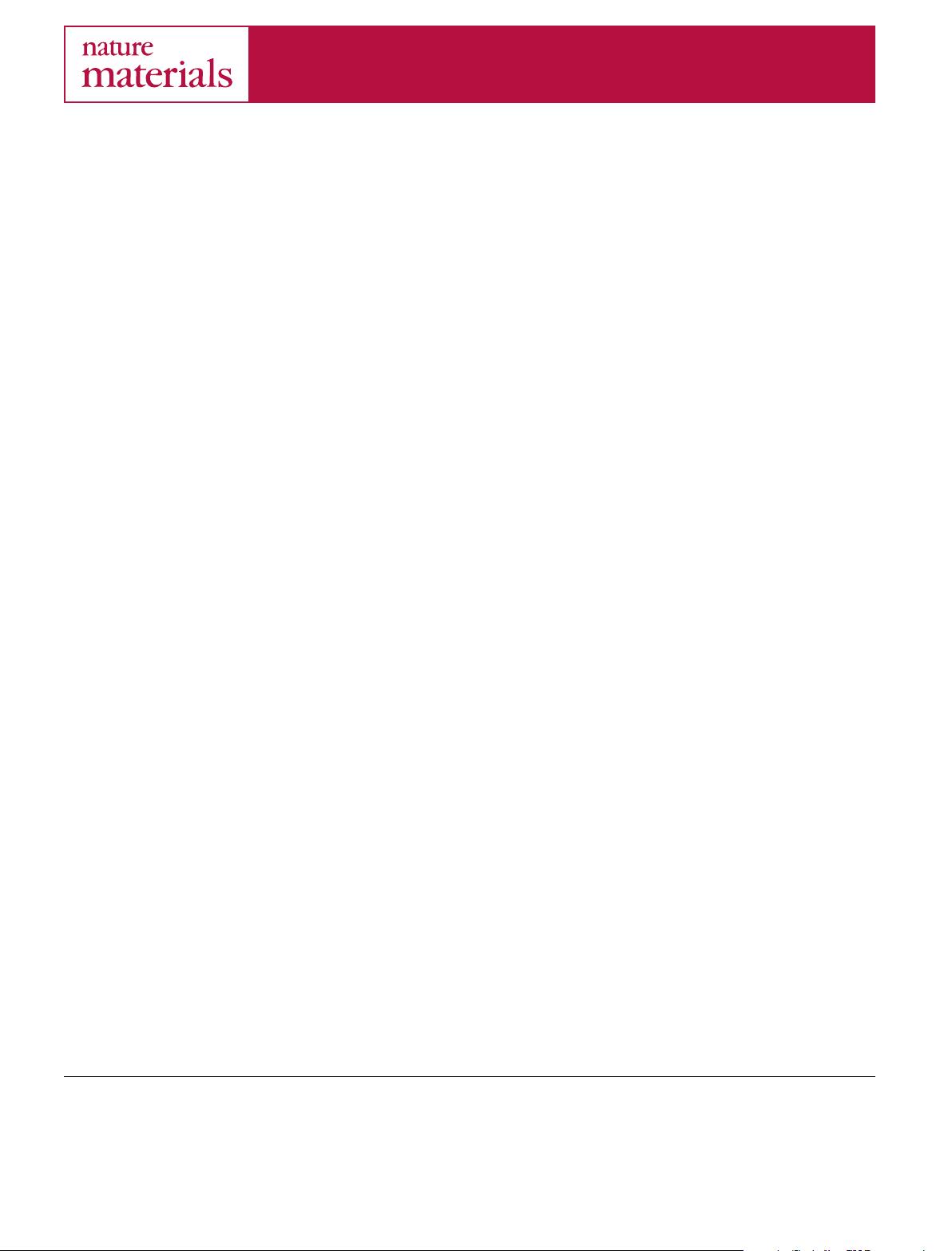
LETTERS
PUBLISHED ONLINE: 12 OCTOBER 2014 | DOI: 10.1038/NMAT4105
Liquid-like pseudoelasticity of sub-10-nm
crystalline silver particles
Jun Sun
1†
, Longbing He
1†
, Yu-Chieh Lo
2,3,4†
, Tao Xu
1
, Hengchang Bi
1
, Litao Sun
1
*
, Ze Zhang
5
*
,
Scott X. Mao
6
and Ju Li
2,3
*
In nanotechnology, small-volume metals with large surface
area are used as electrodes, catalysts, interconnectsand anten-
nae
1–4
. Their shape stability at room temperature has, however,
been questioned. Using in situ high-resolution transmission
electron microscopy, we find that Ag nanoparticles can be
deformed like a liquid droplet but remain highly crystalline
in the interior, with no sign of dislocation activity during
deformation
5,6
. Surface-diusion-mediated pseudoelastic de-
formation is evident at room temperature, which can be driven
by either an external force or capillary-energy minimization.
Atomistic simulations confirm that such highly unusual Coble
pseudoelasticity can indeed happen for sub-10-nm Ag par-
ticles at room temperature and at timescales from seconds
to months.
Nanotechnology requires precise shape control of components.
Once a certain shape is made, it is often hoped that it stays
unchanged over a long period of time. Nanoelectronic
devices with 14 nm feature sizes are commercially available
at present, and we may soon enter the sub-10-nm regime. It
is therefore essential to examine the shape change and shape
stability of sub-10-nm material components, espe cially close to
room temperature.
Although both plastic and pseudoelastic deformations are
used for shaping, and both incur energy dissipation, the essential
difference is that, when un loaded to zero stress, a pseudoelastic
component always recovers to a single ‘rest shape’ regardless of
previous deformation history, whereas a component deformed by
plasticity has no memory of a single rest shape and will stay in the
deformed shape(s) at zero stress (see Supplementary Part 15 for an
illustration of this difference). In t his paper we will show using in situ
high-resolution transmission electron microscopy that sub-10-nm
single crystals of Ag deform pseudoelastically, rather than plastically,
at room temperature. This victory of pseudoelasticity over plasticity
at the small ext remes of size-dependent mechanical behaviour of
metals
5–9
can have three profound practical consequences: there
is no longer ‘plastic freedom’ at the small extremes—that is, the
availability of a large number of arbitrary, stress-free shapes; one
can still gain access to an infinite number of shapes, but different
finite loads must be imposed via external constraints, similar to
inflating a rubbery ballo on; omni-directional, reconfigurable
and damage-tolerant contact is suddenly possible at this
extreme scale.
Liquid-like deformation of silver nanocrystals
Figure 1 shows a typical cycle of compression and stretching of a
silver (Ag) nanocrystal (NC) with a base diameter of 9.8 nm and
partially bonded on the surface of a W tip. The NC height is 4.6 nm
and the shape is stable under electron-beam irradiation during
normal imaging (the beam intensity of 3.8 A cm
−2
is much weaker
than in normal practice)
10,11
(Methods and Supplementary Part 1).
This shape probably possesses the minimum free energy. The facet
shown in Fig. 1a corresponds to the (111) crystal plane of Ag
(Supplementary Part 2). As the NC-decorated W tip approaches the
crystalline ZrO
2
above (Fig. 1b), t he top of the Ag NC snaps slightly
onto the ZrO
2
surface, as a result of van der Waals attraction
12
.
The upper part of the Ag NC is then deformed, as can be seen
in Fig. 1c. As the W tip further approaches the ZrO
2
, compressive
stress is spread onto the entire Ag NC. In Fig. 1d–f, the Ag
NC undergoes drastic deformation, resulting eventually in a flat
pancake-like shape. During the process, the outer morphology of
the Ag NC changes similarly to a liquid drop (Supplementary
Movies 1–3). However, high-resolution images taken during the
process prove that the deforming Ag nanoparticle remains single
crystalline (Fig. 1k,l) inside. When the W tip is detached from
the ZrO
2
(Fig. 1f–i), the Ag NC surprisingly starts to recover its
original shape and the base diameter decreases from 14.3 to 9.5 nm
in the first stage, and then to 9.2 nm. The height of t he Ag NC
recovered quickly and a thin sharp Ag tip meniscus (Fig. 1i) is
formed. When the tip is finally detached, the shape of the Ag
NC changes rapidly from an elongated spire to a st able geometry
with facets similar to its initial ones (Fig. 1j). Such a reversible
change in shape (except for missing atoms induced by effects such
as tip scratching and electron bombardment, which break the ideal
behaviour) is infinitely repeatable (Fig. 1m,n and Supplementary
Movies 1 and 2) just like the deformation of a water droplet, also for
lateral squeezing (Supplementary Part 16) and for smaller Ag NCs
(Supplementary Movie 4). Thus, the videotaped deformations are
pseudoelastic rather than plastic; the memory of a single rest shape
can be explained by capillary-energy minimization—that is, the rest
shape is the result of the balance between Ag surface energy and
Ag–W interfacial energy.
Surface-diusion-dominated Coble pseudoelasticity
Next we demonstrate that the atomic mechanism behind the
shape evolution is surface diffusion. There is prior evidence in the
1
SEU-FEI Nano-Pico Center, Key Lab of MEMS of Ministry of Education, Southeast University, Nanjing, 210096, China,
2
Department of Nuclear Science
and Engineering, Massachusetts Institute of Technology, Cambridge, Massachusetts 02139, USA,
3
Department of Materials Science and Engineering,
Massachusetts Institute of Technology, Cambridge, Massachusetts 02139, USA,
4
Center for Elements Strategy Initiative for Structural Materials (ESISM),
Kyoto University, Sakyo, Kyoto 606-8501, Japan,
5
Department of Materials Science and Engineering, State Key Laboratory of Silicon Materials, Zhejiang
University, Hangzhou 310027, China,
6
Department of Mechanical Engineering and Materials Science, University of Pittsburgh, 3700 O’Hara Street,
Pittsburgh, Pennsylvania 15261, USA.
†
These authors contributed equally to this work.
*
e-mail: slt@seu.edu.cn; zezhang@zju.edu.cn; liju@mit.edu
NATURE MATERIALS | VOL 13 | NOVEMBER 2014 | www.nature.com/naturematerials 1007
© 2014 Macmillan Publishers Limited. All rights reserved