没有合适的资源?快使用搜索试试~ 我知道了~
温馨提示
在质子能量为s = 13 $$ \ sqrt {s} = 13的情况下,进行了在3.2 fb -1质子-质子碰撞中衰变成光子和射流的高质量共振的产生的搜索。 ATLAS探测器在大型强子对撞机上收集的$$ TeV。 选定的事件具有孤立的光子和射流,每个光子和射流的横向动量都超过150 GeV。 没有发现γ+射流不变质量分布与仅背景假设有显着偏差。 通用高斯形信号的横截面和标准模型以外的一些基准现象的极限设置为95%置信水平:激发的夸克与标准模型粒子具有矢量样的耦合,并且在非热量子黑洞中 额外空间维度的两个模型。 高宽比质量比为2%的高斯形共振的最小排除可见截面从质量为1.5 TeV的约6 fb降低为质量为5 TeV的约0.8 fb。 高斯形共振的宽质量比为15%的最小排除可见截面从质量为1.5 TeV的约50 fb降低到质量为5 TeV的约1.0 fb。 对于Randall-Sundrum(Arkani-Hamed-Dimopoulous-Dvali)模型(具有一(六)个额外的尺寸),激动的夸克在4.4 TeV的质量以下被排除,非热量子黑洞不在3.8(6.2)TeV的质量以下。
资源推荐
资源详情
资源评论
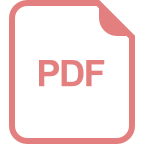
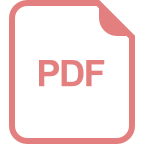
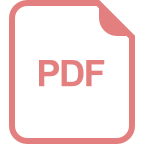
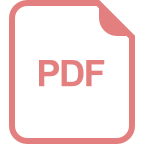
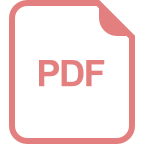
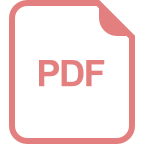
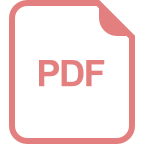
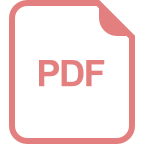
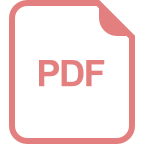
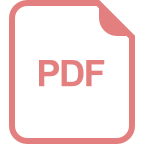
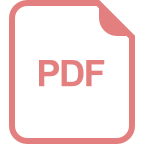
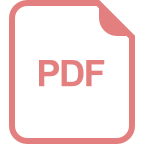
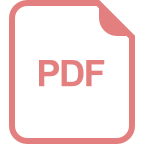
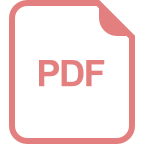
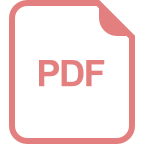
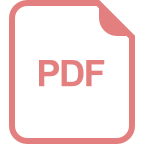
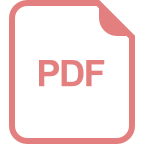
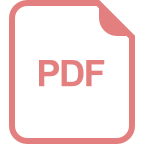
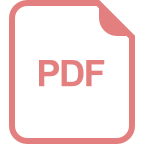
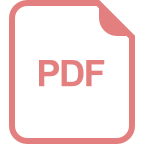
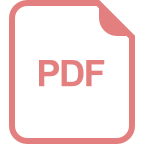
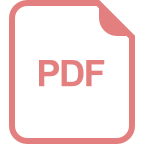
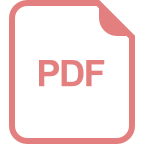
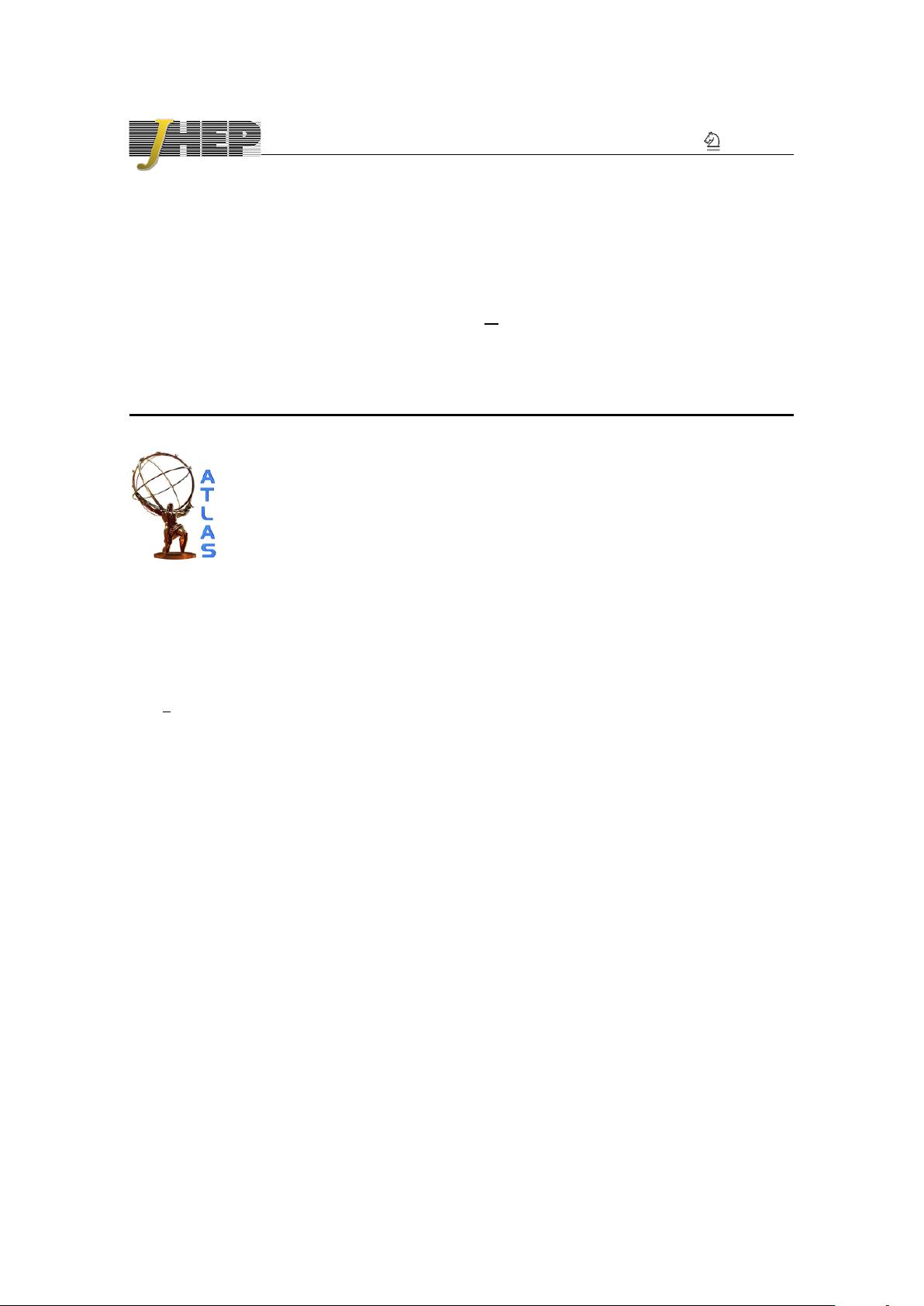
JHEP03(2016)041
Published for SISSA by Springer
Received: December 21, 2015
Revised: January 29, 2016
Accepted: February 17, 2016
Published: March 8, 2016
Search for new phenomena with photon+jet events in
proton-proton collisions at
√
s = 13 TeV with the
ATLAS detector
The ATLAS collaboration
E-mail: atlas.publications@cern.ch
Abstract: A search is performed for the production of high-mass resonances decaying
into a photon and a jet in 3.2 fb
−1
of proton-proton collisions at a centre-of-mass energy
of
√
s = 13 TeV collected by the ATLAS detector at the Large Hadron Collider. Selected
events have an isolated photon and a jet, each with transverse momentum above 150 GeV.
No significant deviation of the γ+jet invariant mass distribution from the background-
only hypothesis is found. Limits are set at 95% confidence level on the cross sections of
generic Gaussian-shaped signals and of a few benchmark phenomena beyond the Standard
Model: excited quarks with vector-like couplings to the Standard Model particles, and non-
thermal quantum black holes in two models of extra spatial dimensions. The minimum
excluded visible cross sections for Gaussian-shaped resonances with width-to-mass ratios
of 2% decrease from about 6 fb for a mass of 1.5 TeV to about 0.8 fb for a mass of 5 TeV.
The minimum excluded visible cross sections for Gaussian-shaped resonances with width-
to-mass ratios of 15% decrease from about 50 fb for a mass of 1.5 TeV to about 1.0 fb for
a mass of 5 TeV. Excited quarks are excluded below masses of 4.4 TeV, and non-thermal
quantum black holes are excluded below masses of 3.8 (6.2) TeV for Randall-Sundrum
(Arkani-Hamed-Dimopoulous-Dvali) models with one (six) extra dimensions.
Keywords: Hadron-Hadron scattering
ArXiv ePrint: 1512.05910
Open Access, Copyright CERN,
for the benefit of the ATLAS Collaboration.
Article funded by SCOAP
3
.
doi:10.1007/JHEP03(2016)041
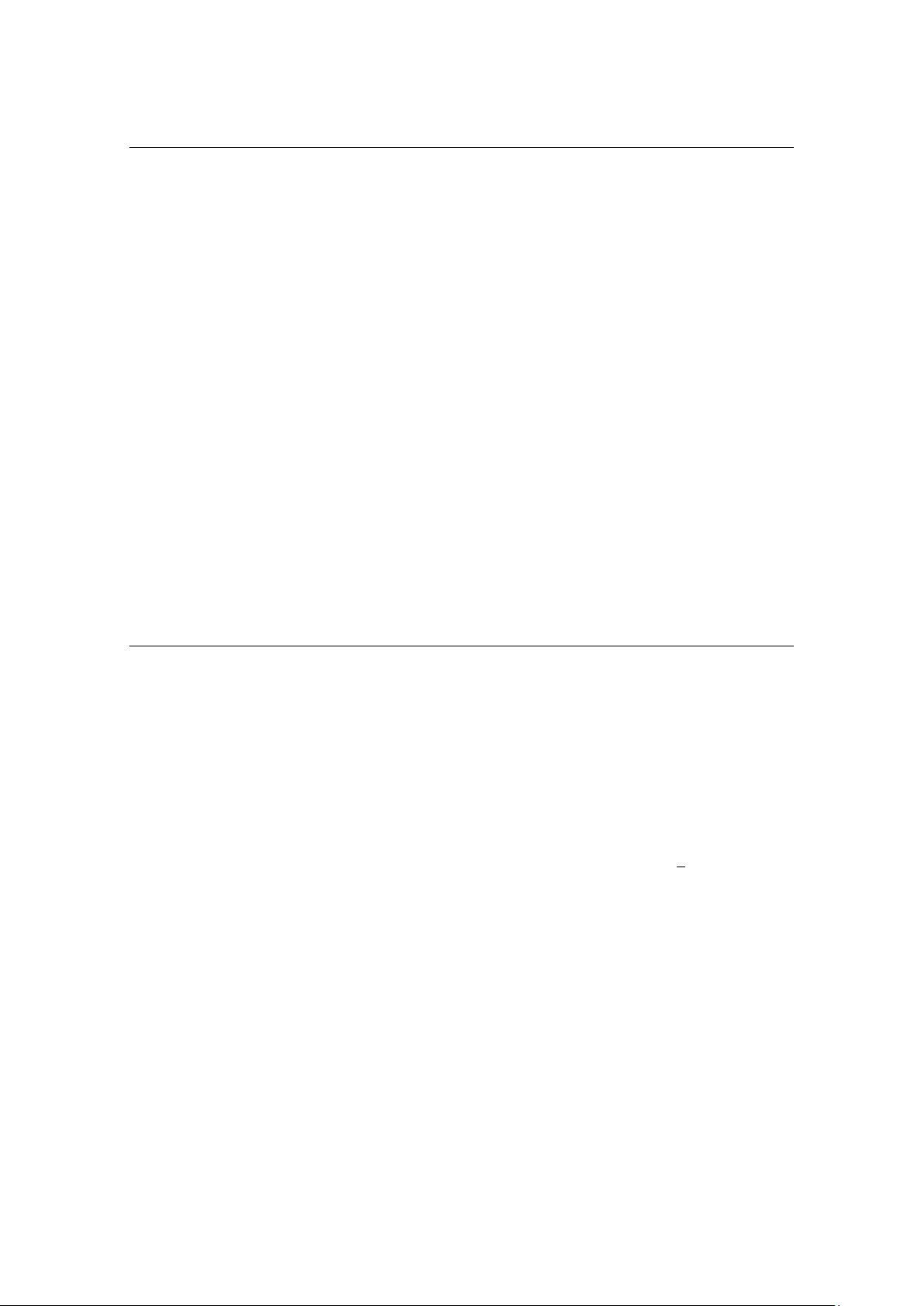
JHEP03(2016)041
Contents
1 Introduction 1
2 The ATLAS detector 4
3 Data and simulation samples 5
4 Event selection 6
5 Signal and background models 8
5.1 Signal model 8
5.2 Background model 10
6 Systematic uncertainties 10
7 Statistical procedures of the excess search 12
8 Results 13
9 Conclusions 13
The ATLAS collaboration 21
1 Introduction
Final states consisting of a photon and a jet (γ + jet) with large invariant mass could be
produced in proton-proton (pp) collisions at the Large Hadron Collider (LHC) in many
scenarios of physics beyond the Standard Model (SM), including decays of excited quarks
(q
∗
) and non-thermal quantum black holes.
Excited-quark states with vector-like couplings to the SM particles [1, 2] may be pro-
duced in pp collisions via the fusion of a gluon with a quark and then decay promptly to a
quark and a photon (qg → q
∗
→ qγ). At a pp centre-of-mass energy of
√
s = 13 TeV, the
expected leading-order (LO) q
∗
production cross sections (pp → q
∗
+ X) times the q
∗
→ qγ
decay branching ratios, combining all flavours of excited quarks and assuming a composite-
ness scale equal to the excited-quark mass m
q
∗
, are shown in figure 1 as a function of m
q
∗
.
These cross sections were obtained with the Pythia 8.186 event generator [3]. Only gauge
interactions like those in the SM are considered for the excited quarks, with the SU(3),
SU(2), and U(1) coupling multipliers fixed to f
s
= f = f
0
= 1. The predicted production
cross section times branching ratio is approximately 5 fb for m
q
∗
= 4 TeV.
Theories with n extra spatial dimensions, such as the Randall-Sundrum type-I (RS1)
model [4] and the Arkani-Hamed-Dimopoulous-Dvali (ADD) model [5, 6], solve the mass
hierarchy problem of the SM by lowering the fundamental scale M
∗
of quantum gravity
– 1 –
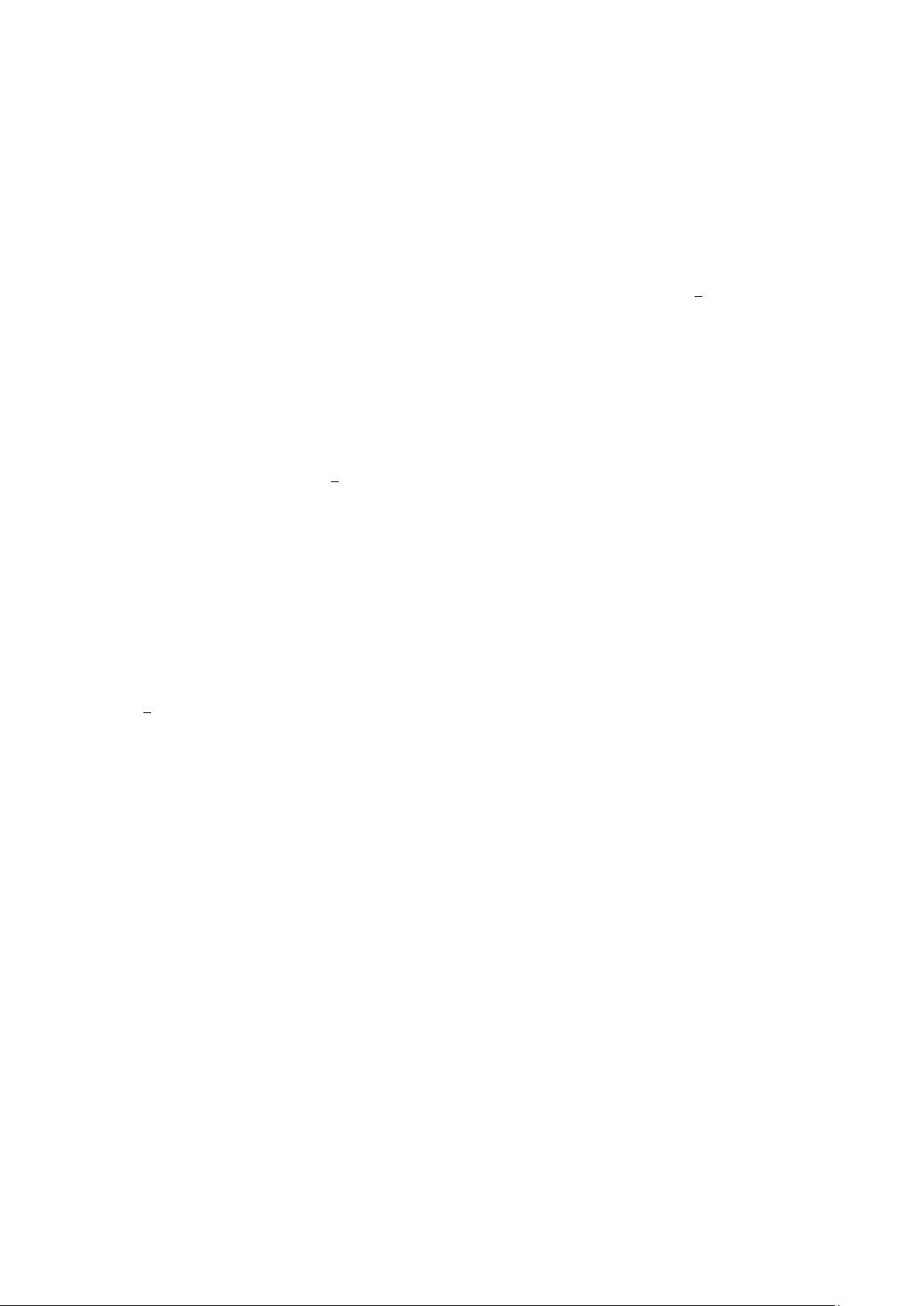
JHEP03(2016)041
(
˜
M in the RS1 model and M
D
in the ADD model) to a few TeV. As a consequence, the
LHC could produce quantum black holes (QBH) with masses near M
∗
[7, 8], which would
then decay before thermalizing, producing low-multiplicity final states [9, 10]. The RS1
model studied in this article has n = 1 extra dimensions. For the ADD model, the same
benchmark scenario (n = 6) is investigated as in the previous ATLAS publication [11]. In
this article it is also assumed that the mass threshold for black hole production is equal to
the Planck scale, M
th
= M
∗
. The maximum mass for black hole production, which in any
case cannot exceed the pp centre-of-mass energy, is set to 3M
∗
(when M
∗
<
√
s/3), to avoid
the high-mass regime in which a classical description of the black hole should replace the
quantum one. A continuum of black holes with invariant masses between the threshold mass
and the maximum mass can therefore be produced, with a probability rapidly decreasing
with the mass. The total expected production cross sections times decay branching ratios
for pp → QBH + X → γ + q/g + X as a function of the threshold mass, assuming that all
QBHs decay to two-body final states and summing over all parton types in the initial and
final state, are shown in figure 1. These cross sections were obtained with the QBH 2.02
event generator [12]. At
√
s = 13 TeV the predicted total production cross section times
branching ratio is 1.4 fb (390 fb) for RS1 (ADD) black holes with M
th
= 4 TeV.
Both the q
∗
→ qγ and QBH → qγ, gγ decays (regardless of the number of extra dimen-
sions) would yield final states with a photon and a jet having large transverse momenta and
large invariant mass m
γj
. Such events would manifest themselves in the m
γj
distribution as
a broad peak above the steeply falling background from SM prompt γ + jet events [13, 14],
typically produced by QCD Compton scattering (qg → qγ).
In this article, a search for a localized, high-mass excess in the γ + jet invariant mass
distribution is presented. The excess would arise from s−channel production of a resonant
signal. The measurement uses 3.2 fb
−1
of pp collisions collected at a centre-of-mass energy
√
s = 13 TeV by the ATLAS detector in 2015.
The results are interpreted in terms of the visible cross section (i.e. the product of the
production cross section, the branching ratio, the detector acceptance and the selection
efficiency) of a generic Gaussian-shaped signal with mass M
G
and width σ
G
. The results
are also interpreted in terms of the cross section times branching ratio to a photon and a
quark or a gluon in three benchmark models: a q
∗
state, a non-thermal RS1 QBH, and a
non-thermal ADD QBH (for n = 6).
For the case of a Gaussian-shaped signal, the width σ
G
is assumed to be proportional
to M
G
; three possible values of σ
G
/M
G
are considered: 2%, 7% and 15%. The experimental
photon+jet invariant mass resolution improves from 2.4% at 1 TeV to 1.5% at 6 TeV. The
smallest value of σ
G
/M
G
(2%) thus corresponds to the typical photon+jet invariant mass
resolution and hence represents the case of an intrinsically narrow resonance.
The RMS width of the q
∗
lineshape is expected to increase from about 250 GeV at
m
q
∗
= 1 TeV to more than 1 TeV at m
q
∗
= 6 TeV and beyond. Quantum black holes are
expected to produce even broader signals, due to the production of a continuum of QBHs
with masses between the threshold mass M
th
and the maximum mass.
Previous searches for generic Gaussian-shaped resonances, excited quarks, and ADD
quantum black holes in the γ + jet final state have been performed by the ATLAS and
– 2 –
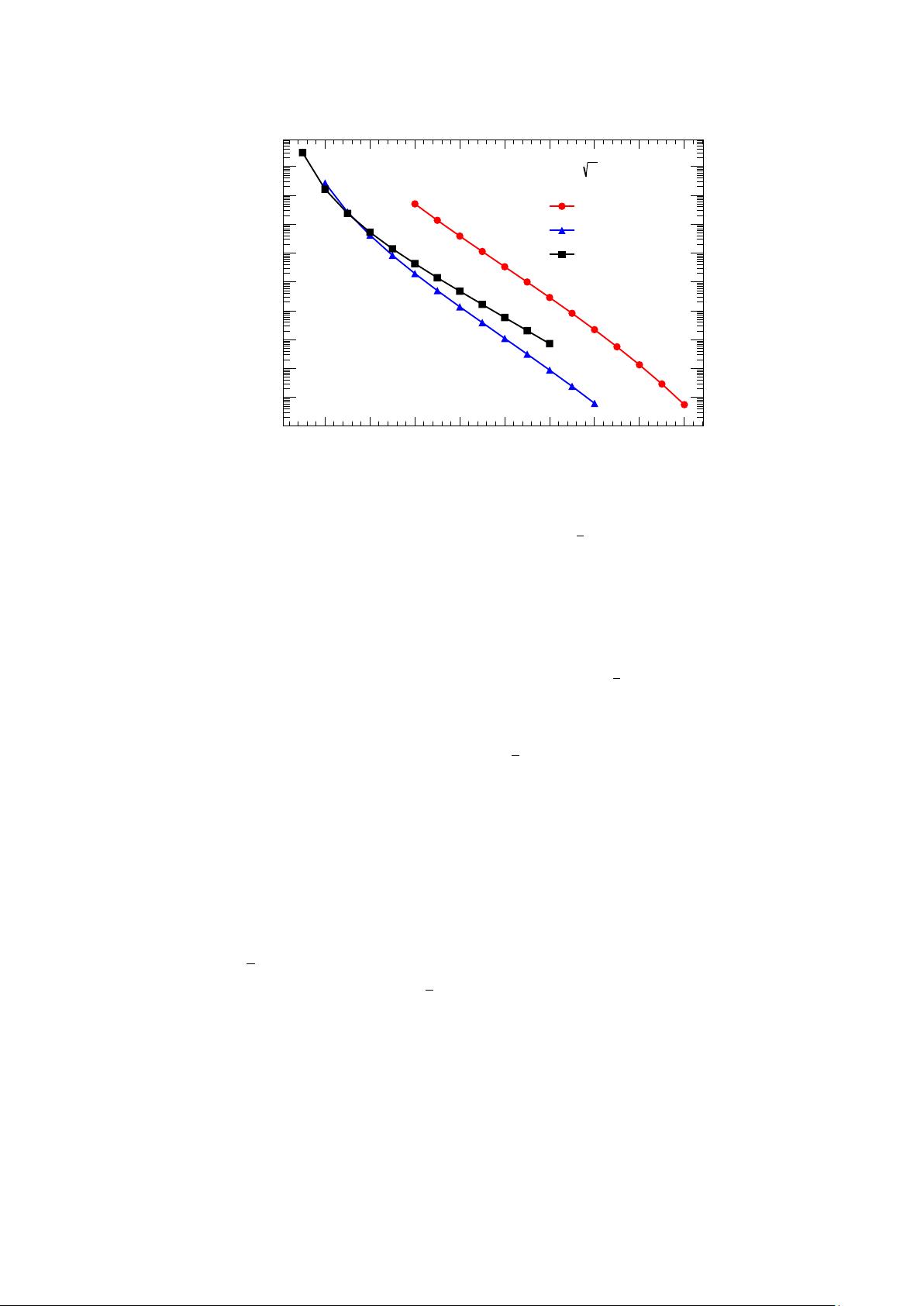
JHEP03(2016)041
[TeV]
q*
or m
th
M
1 2 3 4 5 6 7 8 9
BR [fb]× σ
3−
10
2−
10
1−
10
1
10
2
10
3
10
4
10
5
10
ADD QBH (n=6)
RS1 QBH (n=1)
q*
= 13 TeVs
Figure 1. Production cross section times γ + jet branching ratio for an excited quark q
∗
and two
different non-thermal quantum black hole models (RS1, ADD) as a function of the q
∗
mass or the
mass threshold for black hole production M
th
, in pp collisions at
√
s = 13 TeV. The q
∗
cross section
is computed at leading order in α
s
with the Pythia 8.186 event generator [3]. The excited-quark
model assumes that the compositeness scale is equal to the excited-quark mass m
q
∗
, and that gauge
interactions of excited quarks are like those in the SM, with the SU (3), SU(2), and U(1) coupling
multipliers fixed to f
s
= f = f
0
= 1. The quantum black hole cross sections are obtained with
the QBH 2.02 event generator [12]. In the RS1 and ADD quantum black hole models the number
of extra spatial dimensions is n = 1 and n = 6, respectively. The maximum mass for black hole
production is set to the pp centre-of-mass energy or to 3M
th
if M
th
<
√
s/3. The cross sections are
calculated in 0.5 GeV mass steps (dots) and interpolated with a continuos function (solid lines).
CMS collaborations using pp collisions at either
√
s = 7 TeV [15] or 8 TeV [11, 16]. No
significant excess of events over the background was found, leading to lower limits on the
mass of excited quarks at 3.5 TeV from both the ATLAS and CMS experiments [11, 16]
and on the ADD QBH mass at 4.6 TeV by ATLAS (for n = 6) [11]. No limits on RS1
quantum black holes with the photon+jet final state have been set so far. Using the dijet
final state, ATLAS has set a lower limit on the q
∗
mass at 4.1 TeV and on the ADD QBH
mass at 5.8 TeV for n = 6 [17], while CMS has excluded q
∗
masses below 3.3 TeV and ADD
QBH masses below 4.0-5.3 TeV depending on n [18, 19]. ATLAS has also searched for
quantum black hole production by looking for high-mass dilepton [20] and lepton+jet [21]
resonances in
√
s = 8 TeV data.
Recently, using data collected at
√
s = 13 TeV, the ATLAS and CMS collaborations
performed searches for excited quarks in dijet final states [22, 23], and ATLAS searched
for quantum black holes in dijet final states [22] and for thermal black holes in multijet
final states [24]. Excited quarks with masses below 5.2 TeV and RS1 or ADD (n = 6)
quantum black holes with masses below 5.3 TeV and 8.3 TeV respectively, decaying to
dijets, are excluded.
– 3 –
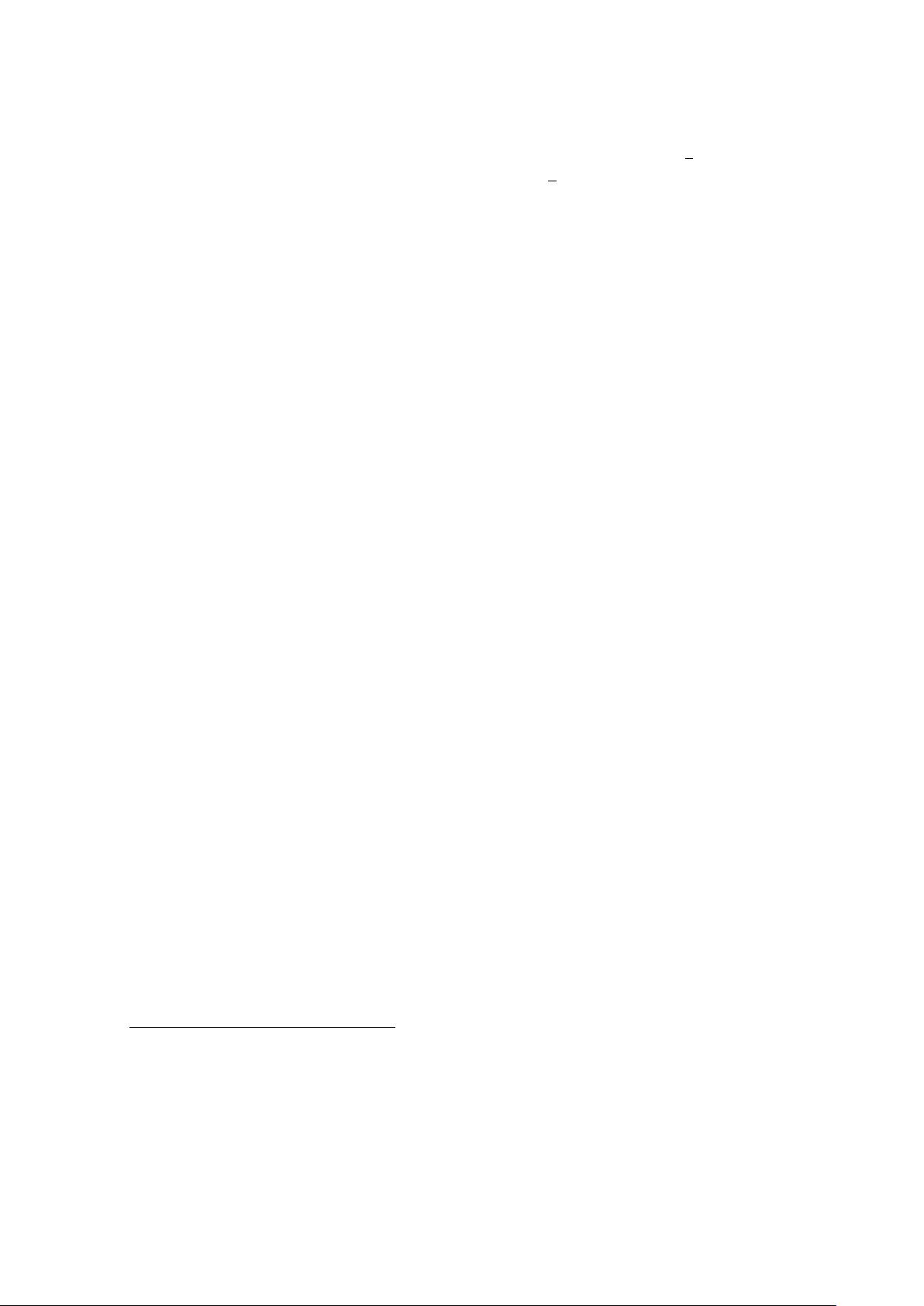
JHEP03(2016)041
The searches presented in this article exploit analysis techniques and a selection strat-
egy similar to those in a previous search using 20.3 fb
−1
of pp collisions at
√
s = 8 TeV [11].
Despite the six times smaller integrated luminosity at
√
s = 13 TeV, the sensitivity of the
present search to the q
∗
and QBH signals exceeds the exclusion limits obtained with 8 TeV
data. This is due to the significant growth of the q
∗
and QBH production cross section
with the increase of the pp centre-of-mass energy from 8 TeV to 13 TeV. For instance, for
a mass of 5 TeV, the production cross sections rise by more than two orders of magnitude
for both the q
∗
and QBHs, while the background cross section increases by less than an
order of magnitude.
The article is organized as follows. In section 2 a brief description of the ATLAS
detector is given. Section 3 summarizes the data and simulation samples used in this
study. The event selection is discussed in section 4. The signal and background modelling
are presented in section 5. The systematic uncertainties are described in section 6. In
section 7, the signal search and limit-setting strategies are discussed, and finally the results
are presented in section 8.
2 The ATLAS detector
The ATLAS detector [25] is a multi-purpose particle detector with approximately forward-
backward symmetric cylindrical geometry.
1
The inner tracking detector (ID) covers |η| <
2.5 and consists of a silicon pixel detector (including the newly installed innermost pixel
layer [26]), a silicon microstrip detector, and a straw-tube transition radiation tracker.
The ID is surrounded by a thin superconducting solenoid providing a 2 T axial magnetic
field and by a high-granularity lead/liquid-argon (LAr) sampling electromagnetic (EM)
calorimeter. The EM calorimeter measures the energy and the position of electromagnetic
showers with |η| < 3.2. It includes a presampler (for |η| < 1.8) and three sampling layers,
longitudinal in shower depth, up to |η| = 2.5. The hadronic calorimeter, surrounding
the electromagnetic one and covering |η| < 4.9, is a sampling calorimeter which uses either
scintillator tiles or LAr as the active medium, and steel, copper or tungsten as the absorber
material. The muon spectrometer (MS) surrounds the calorimeters and consists of three
large superconducting air-core toroid magnets, each with eight coils, a system of precision
tracking chambers (|η| < 2.7), and fast tracking chambers (|η| < 2.4) for triggering.
Events containing photon candidates are selected by a two-level trigger system. The
first-level trigger is hardware based; using a trigger cell granularity coarser than that of
the EM calorimeter, it searches for electromagnetic clusters within a fixed window of size
0.2×0.2 in η×φ and retains only those whose total transverse energy in two adjacent trigger
cells is above a programmable threshold. The second, high-level trigger is implemented in
software and employs algorithms similar to those used offline to identify jets and photon
1
ATLAS uses a right-handed coordinate system with its origin at the nominal interaction point (IP) in
the centre of the detector and the z-axis along the beam pipe. The x-axis points from the IP to the centre
of the LHC ring, and the y-axis points upward. Cylindrical coordinates (r, φ) are used in the transverse
plane, φ being the azimuthal angle around the z-axis. The pseudorapidity is defined in terms of the polar
angle θ as η = − ln tan(θ/2).
– 4 –
剩余36页未读,继续阅读
资源评论
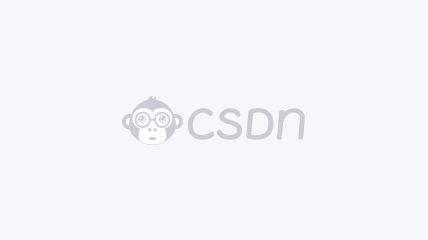

weixin_38685538
- 粉丝: 5
- 资源: 1023
上传资源 快速赚钱
我的内容管理 展开
我的资源 快来上传第一个资源
我的收益
登录查看自己的收益我的积分 登录查看自己的积分
我的C币 登录后查看C币余额
我的收藏
我的下载
下载帮助

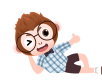
最新资源
资源上传下载、课程学习等过程中有任何疑问或建议,欢迎提出宝贵意见哦~我们会及时处理!
点击此处反馈


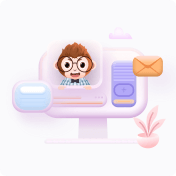
安全验证
文档复制为VIP权益,开通VIP直接复制
