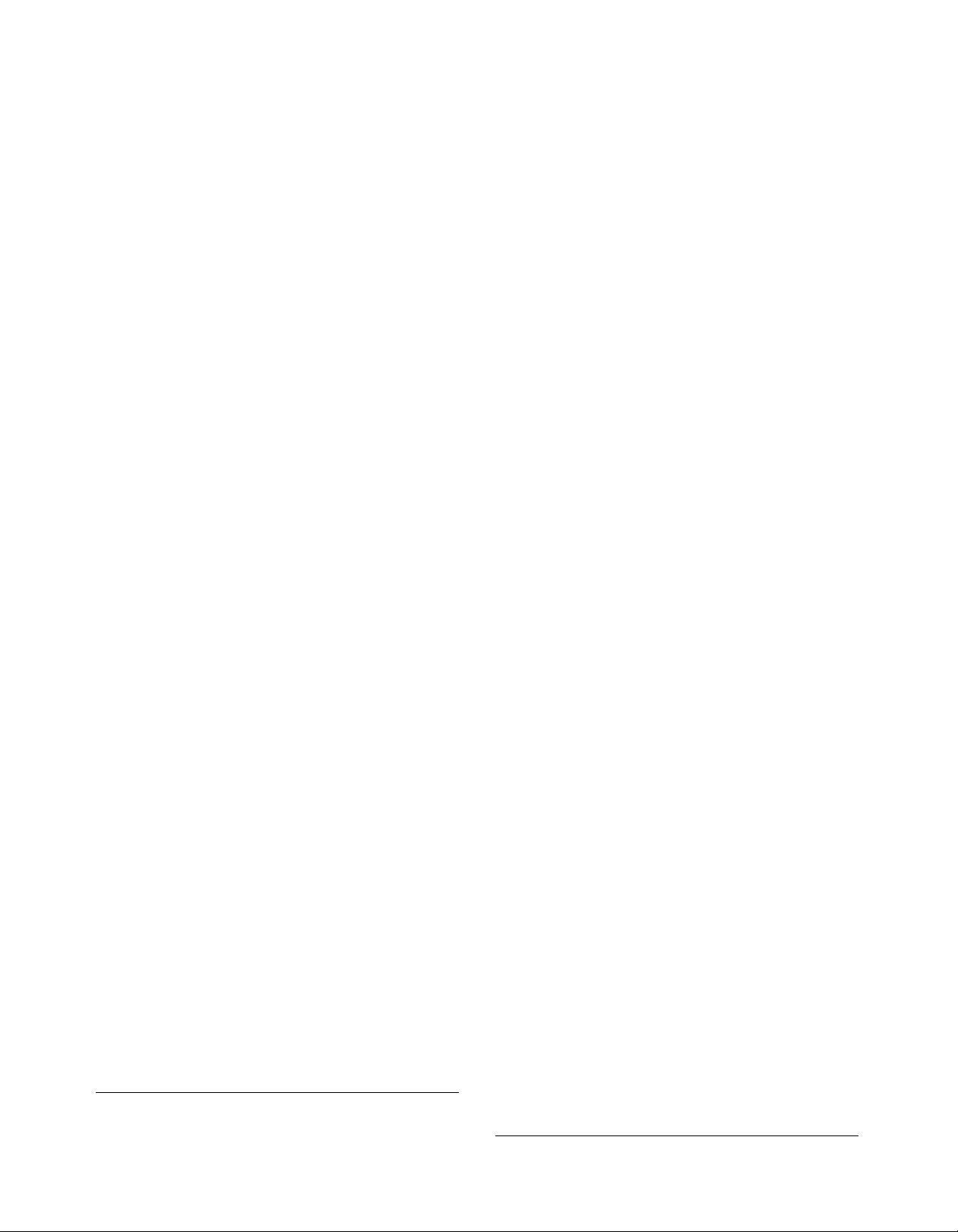
Journal of Biomedical Optics 16(1), 016002 (January 2011)
Multifunctional optical imaging using dye-coated gold
nanorods in a turbid medium
Fuhong Cai,
a,b
Jun Qian,
a,b
Li Jiang,
a,b
and Sailing He
a,b,∗
a
State Key Laboratory of Modern Optical Instrumentation, Zhejiang University, Centre for Optical and Electromagnetic
Research, and Joint Research Center of Photonics of the Royal Institute of Technology (Sweden) and
Zhejiang University, Zijingang Campus, Hangzhou 310058, China
b
Joint Research Laboratory of Optics of Zhejiang University and Zhejiang Normal University, Zhejiang 310058, China
Abstract. We report multifunctional optical imaging using dye-coated gold nanorods. Three types of useful
information, namely, Raman, fluorescence signals, and absorption contrast, can be obtained from a phantom
experiment. These three kinds of information are detected in a nanoparticle-doped-phantom using diffuse optical
imaging. Our novel nanoparticle could be used as a multimodality marker for future bioimaging applications.
C
2011 Society of Photo-Optical Instrumentation Engineers (SPIE). [DOI: 10.1117/1.3526700]
Keywords: diffuse optical imaging; gold nanorods; Raman.
Paper 10159RR received Mar. 24, 2010; revised manuscript received Nov. 5, 2010; accepted for publication Nov. 8, 2010; published
online Jan. 24, 2011.
1 Introduction
Fluorescence imaging with near-IR light is one of the most
common imaging technologies in tissue imaging. Recent nan-
otechnology has greatly accelerated the development of tis-
sue imaging. Several kinds of nanomaterials with specific
optical properties, such as quantum dots (QDs) and upconver-
sion nanoparticles (UCNPs), have been synthesized for tissue
imaging.
1–3
Meanwhile, a gold nanorod (GNR), which is a rod-
shaped type of gold nanoparticle, has many applications, e.g.,
photoacoustic tomography
4
(PAT), photothermal therapy,
5
and
surface-enhanced Raman scattering
6
(SERS). In our paper, dye-
coated GNRs were synthesized and used in a phantom exper-
iment. During our noninvasive scanning of the phantom, three
kinds of information, namely, Raman, fluorescence signals, and
absorption contrast, were obtained for tissue imaging.
Owing to its fingerprint characteristic to molecule struc-
ture, Raman spectroscopy has been well established as a
useful tool for structural characterization and dynamic moni-
toring in various biomedical applications,
7, 8
e.g., Raman mi-
croscopy. Raman spectroscopy has also been studied in the
macro tissue region, such as tissue characterization,
9
Raman
diffuse optical tomography
10
(DOT), and spatially offset Raman
spectroscopy
11
(SORS). There are many advantages to apply-
ing Raman spectroscopy in tissue imaging. First of all, since
each material has its specific Raman spectrum, it is possible to
introduce a Raman signal into an imaging system to suppress
the autofluorescence of the tissue. Second, the unique feature of
the Raman spectrum can help distinguish different exogenous
optical contrast reagents (such as fluorophores) from surround-
ing tissue easily. However, Raman signal is usually too weak to
detect. Some technologies (such as SERS; Refs. 12 and 13)are
used to enhance the intensity of Raman signals to cause them
to reach the same level as that of fluorescence signals. Further-
*Address all correspondence to Sailing He, Joint Research Center of Photon-
ics of the Royal Institute of Technology (Sweden) and Zhejiang University,
East Building No. 5, Zijingang Campus, Hangzhou, Zhejiang 310058, China;
Tel: + 86-571-88206525; Fax: + 86-571-88206512; E-mail: sailing@kth.se.
more, Raman spectra are usually obtained by a “point-to-point”
scanning probe scheme, which is not convenient for measure-
ment in a large area. The original measured Raman spectrum is
usually composed of the fluorescent spectrum and the Raman
“fingerprint” peaks. In a traditional SERS experiment, metallic
structures are adopted to enhance the Raman signals as much
as possible and meanwhile suppress the fluorescent signals by
quenching them to a very low intensity. However, in this paper,
we not only made use of the remaining part of the fluorescence,
which was partly quenched but still detectable during the ex-
periment, but also kept the Raman signals enhanced sufficiently
large for the detection. As both fluorescence and Raman sig-
nals could provide the imaging information of the nanoparticles
inside the phantom, we introduce fluorescence-guided Raman
detection as a new kind of method for Raman measurement. We
make good use of the fluorescent signals, which provide some
additional information for imaging besides Raman signals at
these fluorescent locations for tissue imaging (i.e., in this way it
is not necessary to spend time and effort to study Raman signals
at locations where there are no fluorescent signals).
GNRs have unique optical properties and have attracted
much attention in biomedical applications such as bioimaging,
biosensing, and drug delivery. A GNR has two localized sur-
face plasmon (SPR) resonance (LSPR) bands, which are called
the transverse band and the longitudinal band, corresponding to
electron oscillations in the short and long axes of the nanorod,
respectively. The longitudinal LSPR band expresses strong scat-
tering and absorption and can be tuned from visible to near-IR
(NIR) region by adjusting the aspect ratio (diameter to length) of
the nanorods. GNRs can also be used to enhance the Raman scat-
tering intensity of molecules adsorbed on GNR surfaces up to
10
14
-fold. In this paper, 3,3
-diethylthiadicarbocyanine iodide
(DTDC) and 3,3
-diethylthiatricarbocyanine iodide (DTTC),
which are two kinds of dyes (fluorophores) with rich Raman
spectra, were attached on the surfaces of the GNRs. Under
laser excitation and dye-coated GNRs could exhibit very strong
1083-3668/2011/16(1)/016002/8/$25.00
C
2011 SPIE
Journal of Biomedical Optics January 2011
r
Vol. 16(1)016002-1
Downloaded from SPIE Digital Library on 04 Feb 2011 to 61.175.193.50. Terms of Use: http://spiedl.org/terms