没有合适的资源?快使用搜索试试~ 我知道了~
The surest way to increase the system capacity of a wireless link is by getting the transmitter and receiver closer to each other, which creates the dual benefits of higher quality links and more spatial reuse. In a network with nomadic users, this inevitably involves deploying more infrastructure, typically in the form of microcells, hotspots, distributed antennas, or relays.
资源推荐
资源详情
资源评论
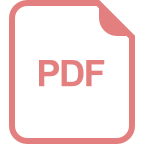
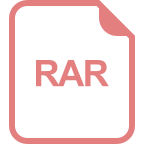
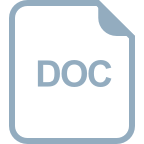
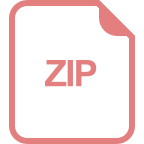
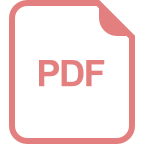
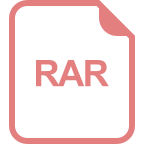
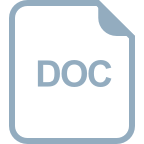
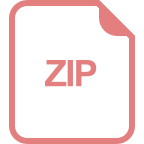
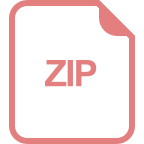
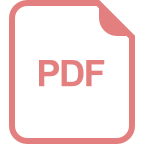
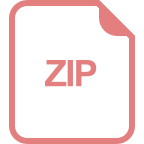
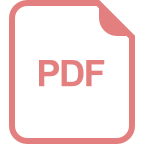
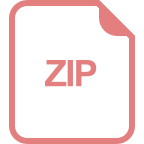
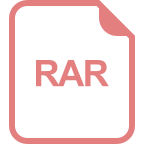
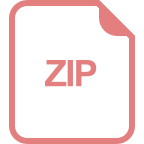
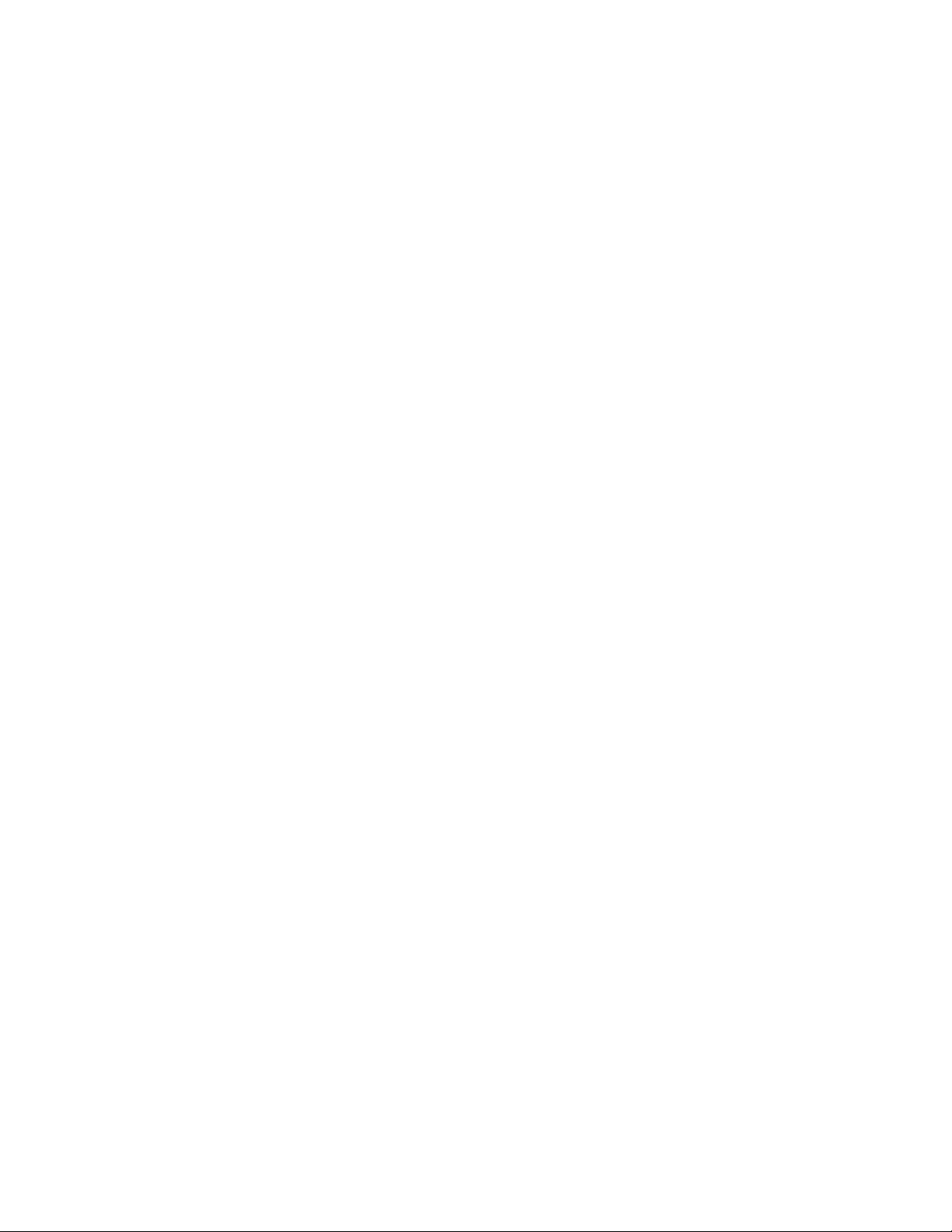
Femtocell Networks: A Survey
Vikram Chandrasekhar and Jeffrey G. Andrews, The University of Texas at Austin
Alan Gatherer, Texas Instruments
June 28, 2008
Contact: jandrews@ece.utexas.edu
Abstract
The surest way to increase the system capacity of a wireless link is by getting the transmitter and receiver
closer to each other, which creates the dual benefits of higher quality links and more spatial reuse. In a
network with nomadic users, this inevitably involves deploying more infrastructure, typically in the form
of microcells, hotspots, distributed antennas, or relays. A less expensive alternative is the recent concept
of femtocells—also called home base-stations—which are data access points installed by home users to
get better indoor voice and data coverage. In this article, we overview the technical and business
arguments for femtocells, and describe the state-of-the-art on each front. We also describe the technical
challenges facing femtocell networks, and give some preliminary ideas for how to overcome them.
1 Introduction
The demand for higher data rates in wireless networks is unrelenting, and has triggered the
design and development of new data-minded cellular standards such as WiMAX (802.16e),
3GPP’s High Speed Packet Access (HSPA) and LTE standards, and 3GPP2’s EVDO and UMB
standards. In parallel, Wi-Fi mesh networks also are being developed to provide nomadic high-
rate data services in a more distributed fashion [1]. Although the Wi-Fi networks will not be able
to support the same level of mobility and coverage as the cellular standards, to be competitive for
home and office use, cellular data systems will need to provide service roughly comparable to
that offered by Wi-Fi networks.
The growth in wireless capacity is exemplified by this observation from Martin Cooper
of Arraycomm: “The wireless capacity has doubled every 30 months over the last 104 years”.
This translates into an approximately million-fold capacity increase since 1957. Breaking down
these gains shows a 25x improvement from wider spectrum, a 5x improvement by dividing the
spectrum into smaller slices, a 5x improvement by designing better modulation schemes, and a
1
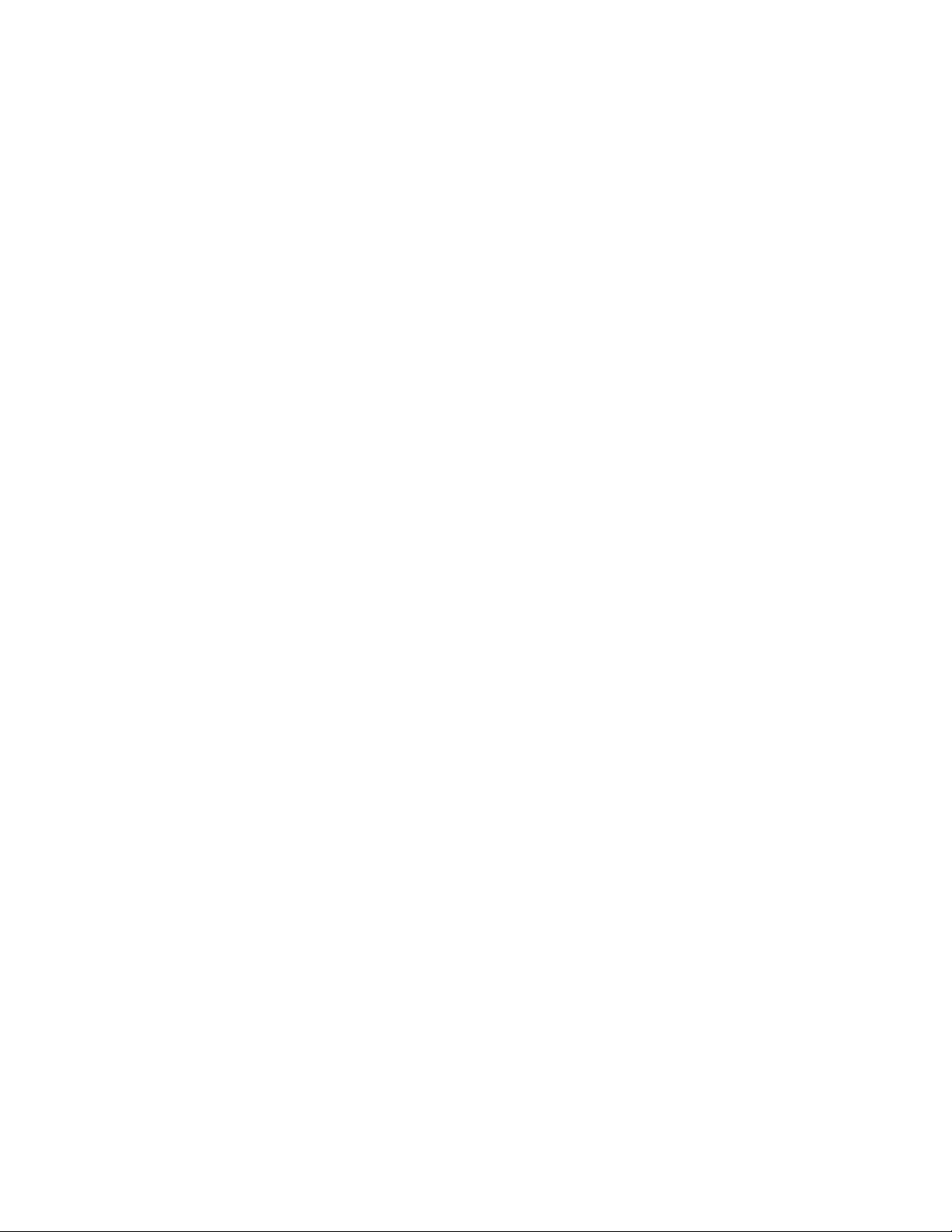
whopping 1600x gain through reduced cell sizes and transmit distance. The enormous gains
reaped from smaller cell sizes arise from efficient spatial reuse of spectrum, or alternatively, a
higher area spectral efficiency [2].
The main problem to this continued micro-ization of cellular networks is that the network
infrastructure for doing so is expensive. A recent development are femtocells, also called home
base-stations, which are short range, low cost and low power base-stations, installed by the
consumer for better indoor voice and data reception. The user-installed device communicates
with the cellular network over a broadband connection such as DSL, cable modem, or a separate
RF backhaul channel. While conventional approaches require dual-mode handsets to deliver
both in-home and mobile services, an in-home femtocell deployment promises fixed mobile
convergence with existing handsets. Compared to other techniques for increasing system
capacity, such as distributed antenna systems [3] and microcells [4], the key advantage of
femtocells is that there is very little upfront cost to the service provider. Table 3 provides a
detailed comparison of the key traits of these three approaches.
Studies on wireless usage show that more than 50% of all voice calls [Airvana, 5] and
more than 70% of data traffic originates indoors [Picochip, 5]. Voice networks are engineered to
tolerate low signal quality, since the required data rate for voice signals is very low, on the order
of 10 kbps or less. Data networks, on the other hand, require much higher signal quality in order
to provide the multi-Mbps data rates users have come to expect. For indoor devices, particularly
at the higher carrier frequencies likely to be deployed in many wireless broadband systems,
attenuation losses will make high signal quality and hence high data rates very difficult to
achieve. This raises the obvious question: why not encourage the end-user to install a short-
range low-power link in these locations? This is the essence of the win-win of the femtocell
approach. The subscriber is happy with the higher data rates and reliability; the operator reduces
the amount on traffic on their expensive macrocell network, and can focus its resources on truly
mobile users. To summarize, the key arguments in favor of femtocells are the following.
Better coverage and capacity. Due to their short transmit-receive distance, femtocells
can greatly lower transmit power, prolong handset battery life, and achieve a higher signal-to-
interference-plus-noise ratio (SINR). These translate into improved reception—the so-called
2
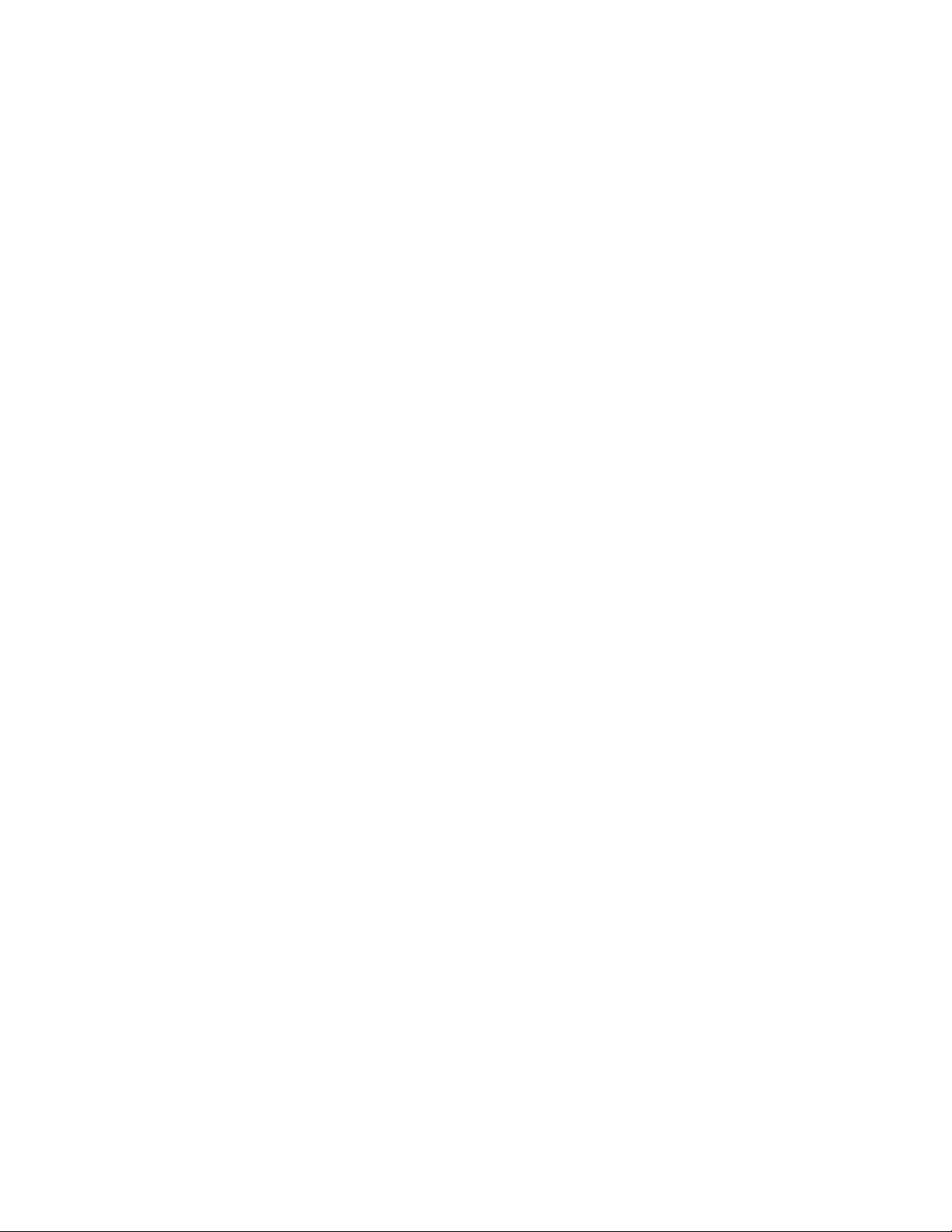
five-bar coverage—and higher capacity. Because of the reduced interference, more users can be
packed into a given area in the same region of spectrum, thus increasing the area spectral
efficiency [2], or equivalently, the total number of active users per Hz per unit area.
Improved macrocell reliability. If the traffic originating indoors can be absorbed into
the femtocell networks over the IP backbone, the macrocell BS can redirect its resources towards
providing better reception for mobile users.
Cost benefits. Femtocell deployments will reduce the operating and capital expenditure
costs for operators. A typical urban macrocell costs upwards of $1K per month in site lease, and
additional costs for electricity and backhaul. The macrocell network will be stressed by the
operating expenses, especially when the subscriber growth does not match the increased demand
for data traffic [Airvana, 5]. The deployment of femtocells will reduce the need for adding
macro-BS towers. A recent study [6] shows that the operating expenses scale from $60K per year
per macrocell to just $200 per year per femtocell.
Reduced subscriber turnover. Poor in-building coverage causes customer
dissatisfaction, encouraging them to either switch operators or maintain a separate wired line
whenever indoors. The enhanced home coverage provided by femtocells will reduce motivation
for home users to switch carriers.
The goal of this article is to provide an overview for these end-user deployed
infrastructure enhancements, and describe in more detail how the above improvements come
about. We also describe the business and technical challenges that femtocells present, and
provide some ideas about how to address them.
2 Technical Aspects of Femtocells
The capacity potential of femtocells can be verified rapidly from Shannon’s law, which relates
the wireless link capacity (in bits/second) in a bandwidth W Hz to the Signal-to-Interference plus
Noise ratio (SINR). The SINR is a function of the transmission powers of the desired and
interfering transmitters, path losses and shadowing during terrestrial propagation. Path losses
cause the transmitted signal to decay as Ad
-α
, where A is a fixed loss, d is the distance between
3
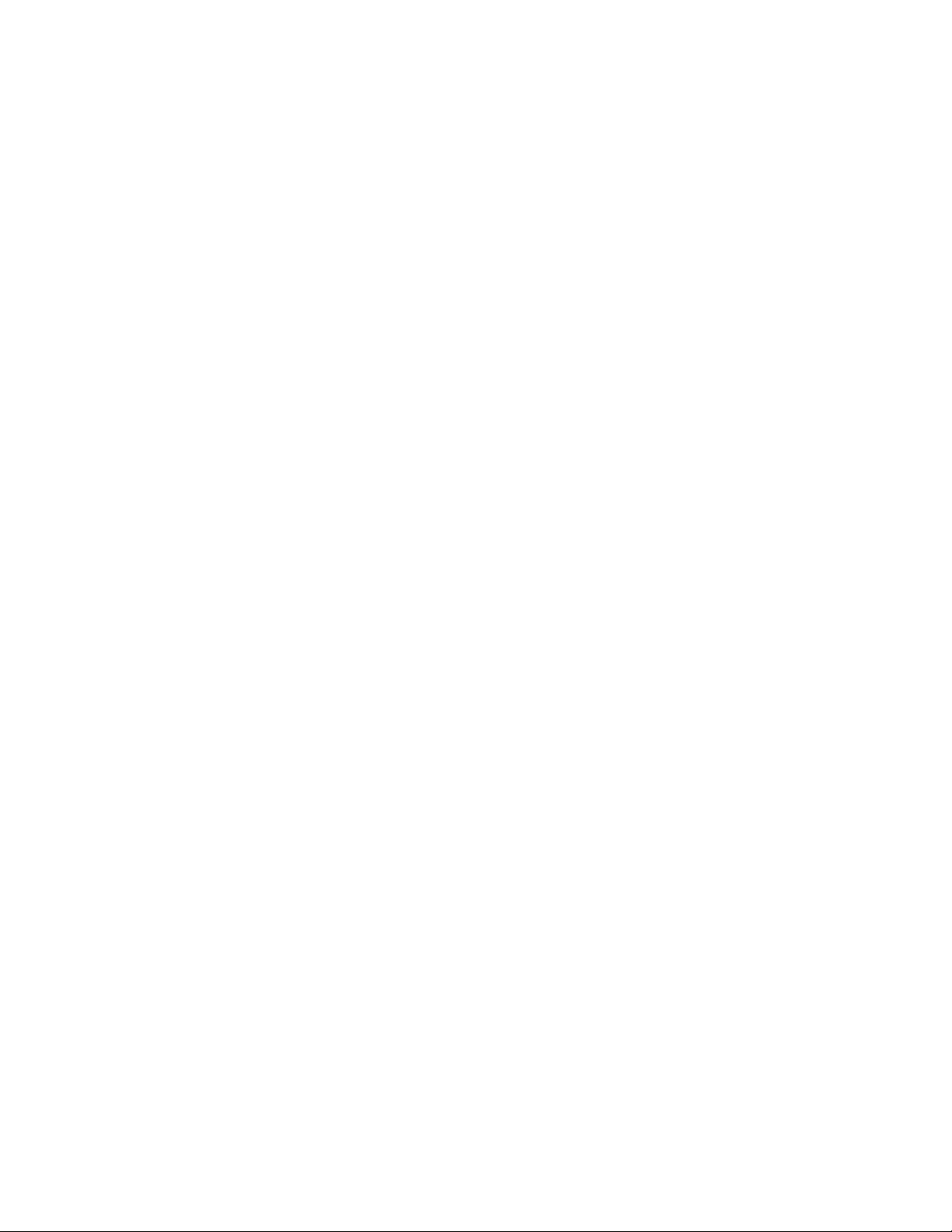
the transmitter and receiver, and α is the path-loss exponent. The key to increasing capacity is to
enhance reception between intended transmitter-receiver pairs by minimizing d and α.
Simultaneously, additional benefits in the network-wide spatial reuse can be obtained by—but
not restricted to—exploiting diversity, and employing interference cancellation, interference
suppression and interference avoidance techniques.
Femtocells enable a reduced transmit power, while maintaining good indoor coverage.
Penetration losses insulate the femtocell from surrounding femtocell transmissions. Assuming a
fixed receive power target with a path-loss propagation model (no fading), and denoting α (resp.
β) as the outdoor (resp. indoor) path-loss exponent, overlaying an area L
2
with N femtocells
results in a transmit power reduction of the order of [10(α-β) log
10
L + 5 β log
10
N] dB.
For
example, choosing a cell dimension of L=1000 meters and N=50 femtocells, with equal path-loss
exponents α=β=4, femtocells give a transmit power saving of nearly 34 dB. When the indoor
path-loss exponent is smaller, say choosing β=2, the transmit power savings increase to nearly 77
dB.
To summarize, the capacity benefits of femtocells are attributed to:
1. Reduced distance between the femtocell and the user, which leads to a higher received signal
strength.
2. Lowered transmit power, and mitigation of interference from neighboring macrocell and
femtocell users due to outdoor propagation and penetration losses.
3. As femtocells serve only around 1-4 users, they can devote a larger portion of their resources
(transmit power & bandwidth) to each subscriber. A macrocell, on the other hand, has a
larger coverage area (500m-1 km radius), and a larger number of users; providing Quality of
Service (QoS) for data users is more difficult.
Points 1 & 2 illustrate the dual improvements in capacity through increased signal strength and
reduced interference. Point 3 shows that deploying femtocells will enable more efficient usage of
precious power and frequency resources. The assumption here is that the wired broadband
4
剩余22页未读,继续阅读
资源评论
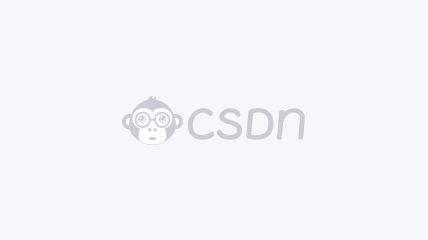

zcqiang2010
- 粉丝: 0
- 资源: 3
上传资源 快速赚钱
我的内容管理 展开
我的资源 快来上传第一个资源
我的收益
登录查看自己的收益我的积分 登录查看自己的积分
我的C币 登录后查看C币余额
我的收藏
我的下载
下载帮助

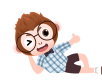
最新资源
- 技术册投标文件的的查重
- 通信原理(第七版 樊昌信 曹丽娜)思维导图
- genad-hGridSample-test.hbm
- cvtocc-shanghai.hbm
- k8s安装ingress-nginx
- dnSpy-net-win32-222.zip
- mongoose-free-6.9
- 德普微一级代理 DP100N06MGL PDFN3.3*3.3 TRMOS N-MOSFET 60V, 8mΩ, 45A
- 【java毕业设计】SpringBoot+Vue幼儿园管理系统 源码+sql脚本+论文 完整版
- 德普微一级代理 DP021N03FGLI DFN5*6 DPMOS N-MOSFET 30V 180A 1.8mΩ
资源上传下载、课程学习等过程中有任何疑问或建议,欢迎提出宝贵意见哦~我们会及时处理!
点击此处反馈


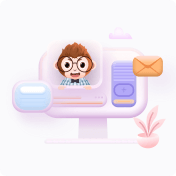
安全验证
文档复制为VIP权益,开通VIP直接复制
