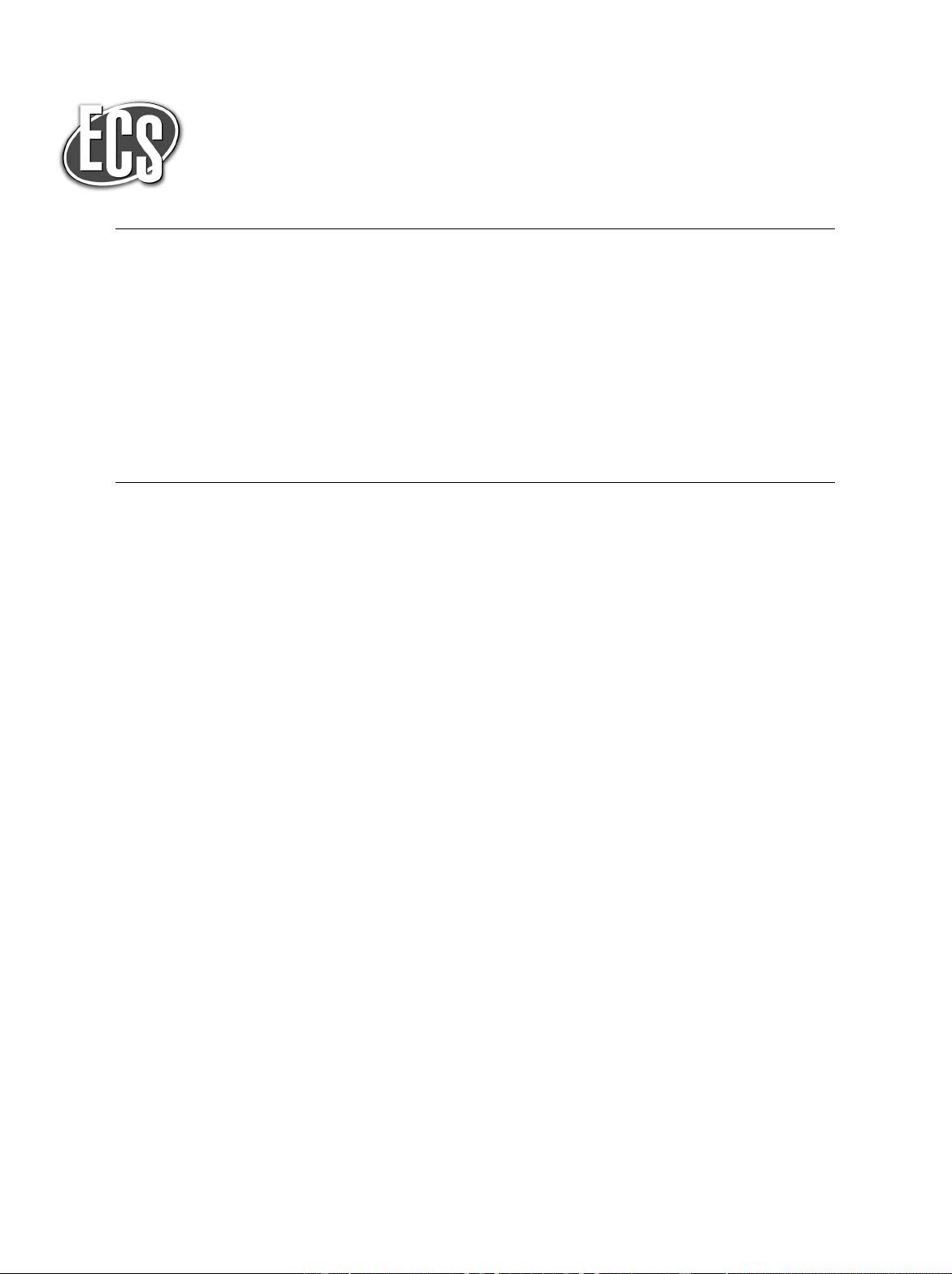
A530 Journal of The Electrochemical Society, 163 (3) A530-A539 (2016)
Na-Ion Desalination (NID) Enabled by Na-Blocking Membranes
and Symmetric Na-Intercalation: Porous-Electrode Modeling
Kyle C. Smith
∗ ,z
and Rylan Dmello
Department of Mechanical Science and Engineering and Computational Science and Engineering Program,
University of Illinois at Urbana-Champaign, Urbana, Illinois 61801, USA
A new device concept, Na-Ion Desalination (NID), is introduced to desalinate NaCl from water using Na-ion battery (NIB)
intercalants. A two-dimensional porous-electrode model is used to predict the performance of NID cells operating at sea- and
brackish-water salinity-levels by simulating electrolyte transport, membrane polarization, and simultaneous electrochemistry. Anion-
selective membranes are used to isolate electrodes in NID cells because simulations showed substantial drop from influent salinity
(63%) compared to porous separators used in conventional NIBs (22%). Symmetric NIB intercalants and energy recovery were
used to minimize energy consumption. A Na
0.44
MnO
2
-based NID-cell with 0.5 mm-thick electrodes desalinated 700 mM influent
by 63% while consuming only 50% more energy (0.74 kWh/m
3
) than thermodynamic minimum when cycled at C/2 rate. The high
volumetric charge-capacity of NIB intercalants enabled 59–64% drop in influent salinity with water-recovery levels up to 80% and
95% for 700 mM and 70 mM influent, respectively. The present predictions of NID performance are optimistic (side reactions,
intercalant decomposition, membrane leakage, and competing cation-intercalation are neglected), providing mechanistic insights
into NID operation that will guide NID development in the future.
© The Author(s) 2016. Published by ECS. This is an open access article distributed under the terms of the Creative Commons
Attribution Non-Commercial No Derivatives 4.0 License (CC BY-NC-ND, http://creativecommons.org/licenses/by-nc-nd/4.0/),
which permits non-commercial reuse, distribution, and reproduction in any medium, provided the original work is not changed in any
way and is properly cited. For permission for commercial reuse, please email: oa@electrochem.org. [DOI: 10.1149/2.0761603jes]
All rights reserved.
Manuscript submitted June 16, 2015; revised manuscript received November 16, 2015. Published January 5, 2016; publisher error
corrected January 21, 2016.
Widespread water scarcity makes cheap, high efficiency desali-
nation systems a global priority.
1
Worldwide installed seawater and
brackish water desalination capacity has been increasing at a rate
of ∼40% per year in the last decade
2
due to population growth,
aquifer shrinkage, and industrial utilization. This increase in world-
wide installed desalination capacity has been driven by reverse osmo-
sis, multi-stage flash, and multiple-effect distillation technologies.
3,4
Electrochemical desalination systems, which include electrodialysis
and capacitive deionization, are also viable technologies that have
been limited to usage in high-recovery and brackish-water desalina-
tion respectively. Capacitive deionization (CDI) was first developed
in the 1960s,
5
and uses porous carbon electrodes to store salt ions in
the electric double-layer to efficiently desalinate brackish water.
6
Re-
cent capacitive-deionization systems use membranes,
7,8
flow-through
electrodes,
9
and hybrid CDI
10
systems to improve desalination per-
formance. Electrodialysis systems, used since the 1960s
11
as a com-
petitor to early reverse osmosis technologies, have improved cycling
efficiency using electrodialysis reversal (EDR),
12
and charge isolation
using porous separators instead of membranes for shielding.
13–15
Recently, devices that induce localized electric fields have
been used to desalinate water, including the ion-concentration
polarization
16
(ICP) and electrochemically mediated desalination
17
(EMD) methods. Similarly, the electric field inside Li-ion batteries
can induce the simultaneous depletion of salt in one electrode and
accumulation in the opposing electrode when cycled at high rate. This
“salt depletion effect” can limit cycling capacity of Li-ion batteries,
18
and consequently energy-storage devices are engineered to prevent
it from occurring. For the present Na-Ion Desalination (NID) cell,
we exploit this effect by blocking Na transport between opposing
electrodes to maximize the degree of salt depletion.
Na-ion intercalation materials have historically been researched
for energy storage.
19
Recent successful demonstrations of Na-ion bat-
teries (NIBs) using NaTi
2
(PO
4
)
3
(NTP, Ref. 20), Na
0.44
MnO
2
(NMO,
Refs. 21,22), Na
2
CuFe(CN)
6
(Ref. 23), and Na
2.55
V
6
O
16
(Ref. 24)
have renewed interest in Na-ion intercalation materials.
25,26
While
capacity and energy density are not as high as in Li-ion batteries,
27
recent developments in NIBs suggest that they can be manufactured
cheaply and electroactive materials can be synthesized by various
∗
Electrochemical Society Active Member.
z
E-mail: kcsmith@illinois.edu
methods.
28–32
Improvements in the capacity and cycle life of NIBs
have also been obtained recently.
33,34,23,24
NIB intercalants have been
used for desalination in hybrid CDI cells in the past.
10
This work
demonstrated the stability of intercalants in seawater and corrobo-
rated earlier attempts to use Na
0.44
MnO
2
in a desalination cell.
35
An
electrochemical cell that uses Na-ion intercalation in both electrodes
(as in the NID cell introduced here) could provide enhanced volu-
metric desalination capacity over a hybrid CDI cell, because Na-ion
intercalants store charge inside electroactive particles while capacitive
desalination only stores charge in the Helmholtz double-layer.
6
To our
knowledge, previously there has been no device proposed to desali-
nate water that uses an electrochemical cell with symmetric electrodes
containing Na-ion intercalants.
We simulate the local electrochemical processes that occur in NID
cells to realistically account for nonlinear and non-uniform polariza-
tion and intercalation effects. With this approach we explore a variety
of cell configurations, comparing between materials, cell dimensions,
and operating parameters to determine the limits of performance
for NID cells. We first describe the materials, system, and porous-
electrode model used presently. The electrochemical processes that oc-
cur during cell cycling are then elucidated by examining the transient
distributions of intercalated Na in electroactive particles and of salt in
the electrolyte. Subsequently, performance is quantified for a range of
applied current density and electrode thickness, after which the influ-
ence of influent salt-concentration and water-recovery is explored.
Methodology
System definition and materials.—Figure 1 shows the two-
dimensional device simulated presently and its dimensions. The NID
device is a symmetric Na-ion cell, meaning that it contains the same
type of Na intercalant in both electrodes (rather than dissimilar in-
tercalants used in conventional NIBs). T hese electrodes are chosen
with common thickness w and length L (Fig. 1b). The electrodes
are porous composites, which enables them to conduct electrons and
ions along the x-direction while saline solution flows through them
along the y-direction (Fig. 1b). The two electrodes can be isolated
(Fig. 1a) by either a polymeric separator (commonly used in NIBs) or
an anion-selective membrane that blocks Na ions.
Next, we describe the operating concept for the present cell. At the
beginning of the charge process electroactive material in the cathode
acts as a source of Na, while electroactive material in the anode
) unless CC License in place (see abstract). ecsdl.org/site/terms_use address. Redistribution subject to ECS terms of use (see 182.72.186.54Downloaded on 2016-02-04 to IP