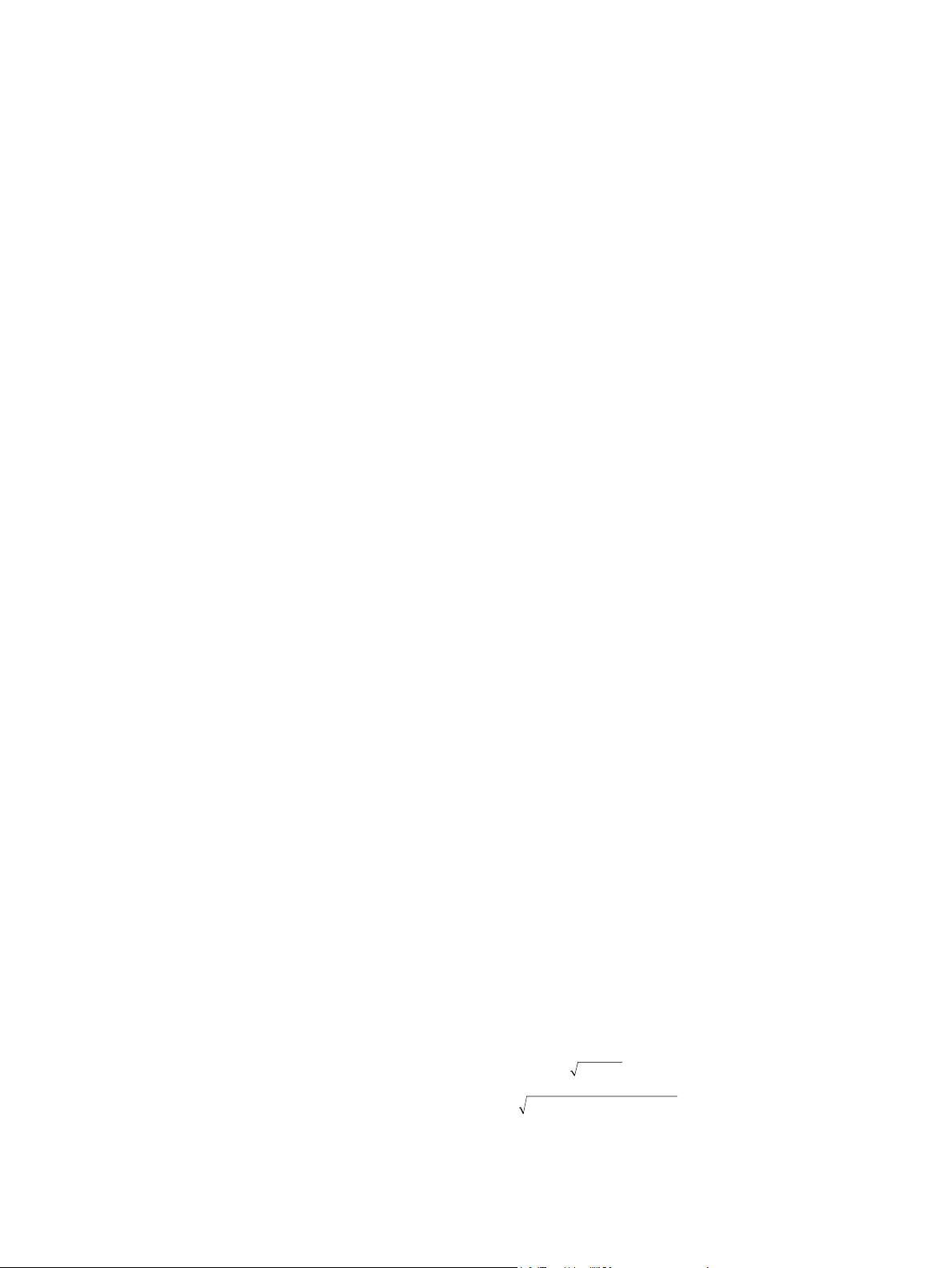
sequence [12,13]. A technique combining simultaneous multi-slice
(SMS) imaging with the RF-spoiled GRE sequence also has been suc-
cessfully applied in temperature imaging [9,14]. However, these se-
quences have limitations in SNR or acquisition time to achieve the best
temperature uncertainty performance [15] and a higher temporal re-
solution [9]. Moreover, a large field of view (FOV) for temperature
imaging is required to monitor the unwanted heating in the tissue in-
terface and the area far from the focus of HIFU heating [16,17], which
requires a long acquisition time.
Multi-channel surface coil arrays [18] placed in close contact with
subjects, capable of high SNR and high parallel imaging capacity, can
quickly produce high-resolution images with accurate temperature es-
timation, especially the dedicated coil arrays for different MR-guided
HIFU applications [19–22]. In turn, faster acquisitions using multi-
channel coils can reduce artifacts from subject motion.
Before implementing HIFU therapy to patients using new fast ima-
ging technologies, such as new sequences, temperature mapping of
animals is generally used for safety testing. Rabbit experiments are the
commonly implemented, such as on rabbit leg muscle [23,24]. How-
ever, in these HIFU therapy studies, the single surface coils were ap-
plied to receive signals, and were unable to take advantage of image
acceleration techniques. For example, studies were limited in SMS and
parallel imaging in fast MRT.
In conjunction with the fast 3D MR thermometry technology [25], a
dedicated RF coil array for MR-guided HIFU studies on rabbit leg
muscle at a 3 T MRI scanner is required. The receiver coil array needs to
be flexible, so as to be placed proximally to the rabbit for better SNR.
Moreover, to ensure the sensitivity maps with sufficient variations
around the ROI, the coil array needs to be designed with a high element
density and an appropriate element arrangement. Combining the
dedicated coil array with the fast 3D MR thermometry based on an
echo-shifted sequence with parallel acquisition [25], temperature
measurements with large coverage and high temporal resolution can be
performed. These animal experiments can demonstrate potential clin-
ical applications using this fast imaging technology for temperature
mapping in MR-guided HIFU treatments.
In this study, a flexible 9-channel coil array was designed and built
for fast 3D MR thermometry in MR-guided HIFU studies on a 3 T MRI
system (TIM Trio, Siemens, Erlangen, Germany). Compared to a com-
mercially available 4-channel coil array (Flex Small 4, dimensions:
366 mm × 174 mm, Siemens, Erlangen, Germany), which is the most
applicable coil for MR-guided HIFU studies on rabbits among all the
commercial coils because of its suitable size and layout, the dedicated
9-channel coil array for MR-guided HIFU studies on rabbit leg muscle
achieved better SNR and parallel imaging, and has improved tem-
perature measurement accuracy.
2. Methods
2.1. Coil construction
We constructed a flexible 9-channel coil array that consists of an 8-
channel coil array to cover the top of the rabbit leg and a single loop on
the bottom of the rabbit leg for the HIFU path. Fig. 1a shows the layout
and size of the flexible 9-channel coil, and the photograph of the coil
array is shown in Fig. 1b). The design principles are described as fol-
lowing.
The channel count and the element size should be chosen reason-
ably to achieve a high SNR [26–28].
Different coils with various sizes
can produce different imaging depths [28]. To avoid pathway inter-
ference of the ultrasound, a single circle loop with a diameter of 60 mm
was placed on the hind rabbit leg to facilitate HIFU heating experiments
on rabbit leg muscle at a depth of about 20 mm. If only a single surface
coil was used, the SNR improved only over a limited region, and fast
imaging with parallel acquisition could not be applied. Therefore, for
fast temperature mapping on rabbits with weight ranging from 3 kg to
5 kg, and based on the SNR contribution of the coil size at an imaging
depth of about 65 mm from the top of the rabbit, a flexible 8-channel
coil array covering the top of the rabbit leg was designed to achieve a
high parallel imaging capacity in temperature mapping with an accel-
eration factor of 2 × 2 in the Antero-Posterior (AP) direction and Left-
Right (LR) direction. The spacing between the 8-channel coil array and
the rabbit was about 5 mm, which was maintained by the coil cover
made in polyethylene material. The single loop was cover by an in-
sulated and heat resistant polymer material, resulting in a spacing of
1 mm between the single loop and the rabbit.
Because there was a certain distance of about 40 mm between the
single coil and the nearest neighboring elements of the 8-channel coil
array, the coupling was not very large. To sufficiently decouple the
single loop and the other array elements, we only applied the decou-
pling method of employing low input-impedance preamplifiers [18].
For the decoupling of the 8-channel coil array, different methods were
applied, including the overlapped decoupling method for the adjacent
elements [18], employment of low input-impedance preamplifiers [18],
and connecting two overlapping self-made inductances for the next
nearest neighboring elements that had a distance of about 20 mm [29].
The overlapping self-made inductances were made into a flat solenoid
shape to achieve a good decoupling and a convenient adjustment, as
depicted in the enlarge picture in Fig. 1b.
Fig. 2 shows the circuit schematics of the element of the 8-channel
coil and the single channel loop. With tuning and matching circuits,
each element was tuned to 123.2 MHz by modifying the capacitors C0
(JR400, VOLTRONICS), and 50 Ω impedance-matched by modifying
the capacitor C2 (100B, Dalian Dalicap Technology Corporation (DLC),
Liaoning, China) in the coil loop. Preamplifier decoupling was achieved
by adjusting the capacitor C3 (JR400, VOLTRONICS) and C4 (100B,
DLC), which were welded on the preamplifier board. One may repeat
the adjustment of the capacitors C2 and C3 until the tuning, matching,
and preamplifier decoupling requirements are met. To decouple the
transmitted coil and the received coil, an active-detune circuit in-
cluding a PIN diode (DH80055-40 N, TEMEX) and an inductance L1 was
added in parallel to the capacitor C1, as shown in Fig. 2. The PIN diode
was biased with an about 100 mA current during the transmit mode,
and was reversed biasing with nearly −30 V during image acquisition.
To minimize the RF interference from the power supply of the PIN
diode, an RF choke was added in series with the biasing line, external to
the coil, which was an inductance with a value of 2.7 μH (1008CS-
272XJLD, Coilcraft).
2.2. In-vivo studies
The animal in-vivo studies were approved by the animal ethics
committee of Shenzhen Institutes of Advanced Technology, Chinese
Academy of Sciences. For the in-vivo thermometry experiments, the
rabbit was placed on an acrylic container, and an MR-compatible,
single-element HIFU transducer (IMASONIC, Besancon, France) with
the
parameter of focal length/diameter = 10 cm/10 cm at 0.8 MHz, was
placed in the water box directly under the container. In order to se-
parate the rabbit from water in the box, a thermoplastic polyurethane
(TPU) membrane covered the hole at the bottom of the container. Fig. 3
illustrates the HIFU heating in-vivo experiment setup.
The coil performance was evaluated by the covariance weighted
SNR and SENSE g-factor maps [30–32]. The formulas are as follows:
=
−−
SNR P PΨ
rSoS Hcov 1
(1)
=⋅
−− −
gSSSS[(Ψ)](Ψ)
ρ
H
ρρ
H
ρρ
11
,
1
,
(2)
wherein P is the signal image, H indicates transposed complex con-
jugate, Ψ denotes the noise matrix, and S corresponds to reduced
Fourier encoding with an acceleration factor R. The g-factor is a
mathematical function of the coil sensitivities and the acceleration
Q. Chen, et al.
Magnetic Resonance Imaging 65 (2020) 37–44
38