Chemistry in Catalysis
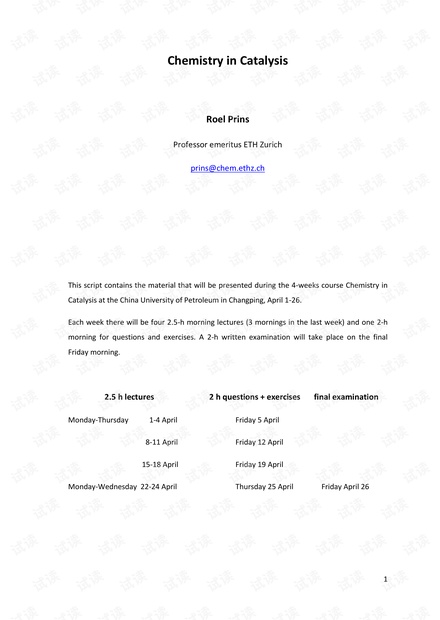

3 4.6 Carbon 108 4.7 Preparation of supported catalysts 109 5. Kinetics 5.1 Langmuir-Hinshelwood model 115 5.2 Monomolecular reaction 117 5.2.1 Surface reaction rate determining 118 5.2.2 Adsorption of A rate determining 121 5.2.3 Desorption of B rate determining 121 5.3 Bimolecular reaction 121 5.4 Influence of diffusion 122 6. Metal Surfaces 6.1 Surface structure 130 6.2 Surface analysis 134 6.2.1 XPS 135 6.2.2 Surface sensitivity 139 6.2.3 Auger electron spectroscopy 140 6.3 Surface enrichment 142 6.4 Metal binding 147 7. Metal Catalysis 7.1 Dissociation of H 2 154 7.2 Hydrogenation of ethene 158 7.3 Ammonia synthesis 162 7.4 CO hydrogenation 7.4.1 Fischer-Tropsch and Methanation reaction 166 7.4.1.1 CO dissociation 167 7.4.1.2 Methanation 172 7.4.1.3 Fischer-Tropsch reaction 1752 1. Introduction p. 1.1 Kinetics and thermodynamics 5 1.2 Activity, selectivity and stability 8 1.3 Heterogeneous, homogeneous and enzymatic catalysis 10 2. Adsorption 2.1 Physisorption 13 2.1.1 Adsorption on surfaces 16 2.1.2 Adsorption isotherms 18 2.1.3 Multilayer adsorption, BET 20 2.2 Surface Diffusion 26 2.3 Chemisorption 2.3.1 Chemical bonding 29 2.3.2 Dissociative chemisorption 37 3. Catalyst Characterization 3.1 Crystal structures 43 3.2 X-ray diffraction 51 3.3 Electron Microscopy 55 3.4 Scanning tunneling microscopy and atomic force microscopy 61 3.5 Infrared and Raman spectroscopy 64 3.6 Nuclear magnetic resonance 74 3.7 Temperature-programmed techniques 79 4. Catalyst preparation 4.1 Heterogeneous catalysts 83 4.2 Alumina supports 86 4.3 Surface of -Al 2 O 3 93 4.4 Silica 97 4.5 Zeolites 1007.4.2 Methanol synthesis 178 7.5 Volcano curves 183 7.6 Brønsted-Evans-Polanyi relations 186 8. Catalysis by Acids 8.1 Reactions catalyzed by solid acids 197 8.2 Reactions of carbenium Ions 199 8.3 Applications of carbenium Ions 207 8.4 Bifunctional catalysis 8.4.1 Principles 209 8.4.2 Industrially relevant bifunctional catalysis 218
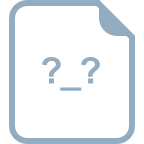
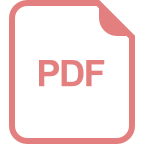
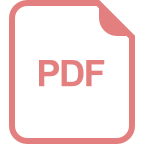
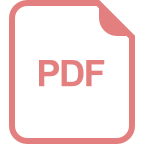
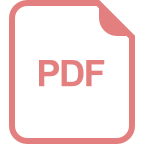
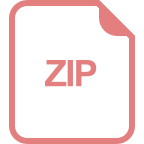
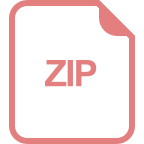
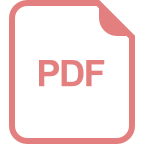
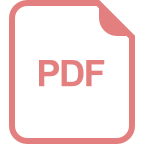
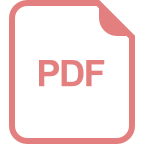
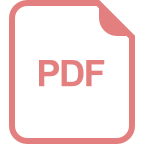
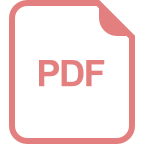
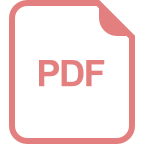
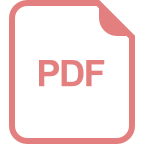
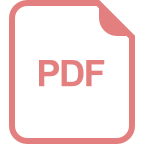
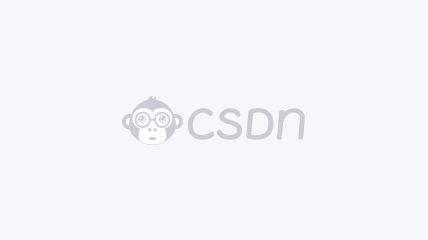

- 粉丝: 0
- 资源: 1
我的内容管理 展开
我的资源 快来上传第一个资源
我的收益
登录查看自己的收益我的积分 登录查看自己的积分
我的C币 登录后查看C币余额
我的收藏
我的下载
下载帮助

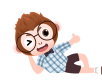
