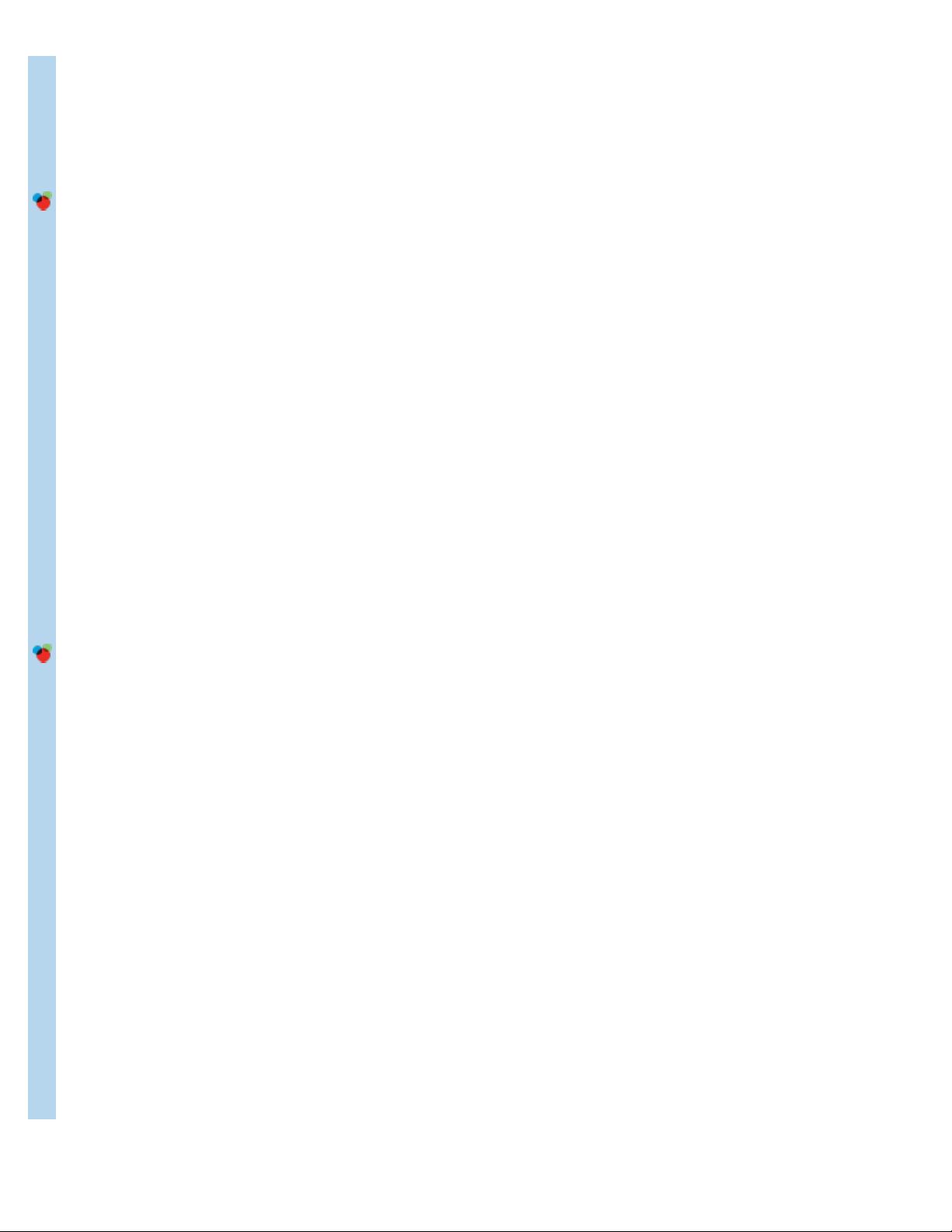
1.
When introducing an artificial synapse, the current entering the post-synaptic cell
depends on the membrane potential of the pre-synaptic neuron by virtue of the
voltage-dependent transmitter release and on the membrane potential of the post-
synaptic target cell through Ohm’s law. Accordingly, in this experiment, two
neurons are monitored intracellularly and one of them, the designated post-synaptic
cell of the simulated synapse receives current injections. The current through the
simulated synapse is calculated from the measured pre- and post-synaptic
membrane potentials using a computational model. StdpC provides two common
computational models for chemical synapses; the one used for our example dataset
(see below) is illustrated in Box 1.
2.
When introducing a simulated ion channel into a target neuron, only the target
neuron’s membrane potential needs to be measured and current injections occur
into the same cell. The computational model in this case is based on Hodgkin-
Huxley-type equations. StdpC provides two common formalisms to parameterise
the equations. The formalism used in the anticipated results (below) is illustrated in
the supplementary equations.
3.
In pattern clamp, both a simulated neuron and a simulated strong gap junction are
used. A target cell is monitored and its membrane potential is clamped to the
activity pattern of the membrane potential of the simulated neuron by the simulated
gap junction.
In the anticipated results section we show examples of these three types of experiments
using either neurons from a classical molluscan preparation or neurons from dissociated
hippocampal cultures.
For designing new dynamic clamp experiments, or adapting existing ones, the experimenter
needs to identify which measurements need to be taken, which neurons need to receive
current injections and what the magnitudes of the measured signals and injected currents are.
When using a single recording/injecting electrode, it is important to reduce any artefact in
the readout of the voltage that may be caused by the simultaneous current injections (see
step 4 in the procedure for a list of options). The voltage drop across an uncompensated
electrode is proportional to the electrode resistance and the amplitude of the injected current
and becomes problematic in procedures that require large current injections such as pattern
clamp. The voltage artefact is absent when no current is injected; for this reason no
compensation is required when using two different electrodes for voltage recording and
current injection. On the other hand, injection artefacts can typically be ignored when the
resistance of the electrode is low (e.g., in patch clamp) and the current injection amplitudes
are small.
Other parameters that need to be taken into account when designing experiments are the
maximum number of electrodes and manipulators that can be used at once on a preparation
and the number of input and output channels available on the data acquisition board used for
dynamic clamp. For example, the Digidata 1200/A boards have two analog outputs whereas
the NI PCI-6229 card we used here has four. The number of analog inputs is typically less
problematic. The Digidata 1200/A has 16 while the NI PCI-6229 board has 32. Along with
the practical experimental considerations, another important consideration in designing
dynamic clamp experiments is to formulate or obtain an
accurate
computational model of
the simulated electrical component. For example, for simulating ion channels, a Hodgkin-
Huxley type model with accurate parameter values is required or, for simulating a synapse, a
full description including conductance, time scale, activation characteristics and reversal
potential is necessary.
Kemenes et al.
Page 4
Nat Protoc
. Author manuscript; available in PMC 2011 October 06.
Europe PMC Funders Author Manuscripts Europe PMC Funders Author Manuscripts