没有合适的资源?快使用搜索试试~ 我知道了~
The structural basis for 2′−5′:3′−5′-cGAMP synthesis by cGAS.pdf
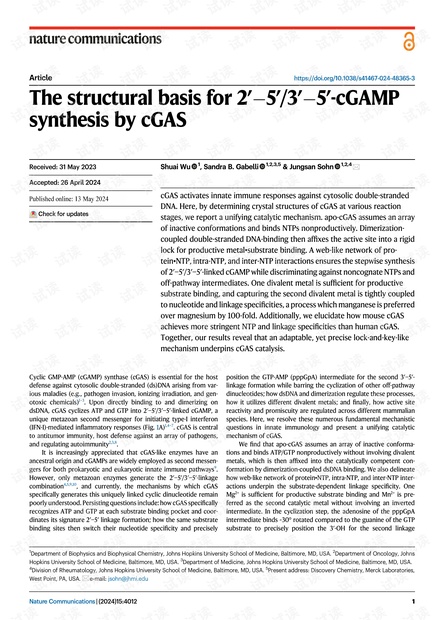

试读
13页
需积分: 0 1 下载量 79 浏览量
更新于2024-10-15
收藏 3.44MB PDF 举报
The structural basis for 2′−5′:3′−5′-cGAMP synthesis by cGAS.pdf
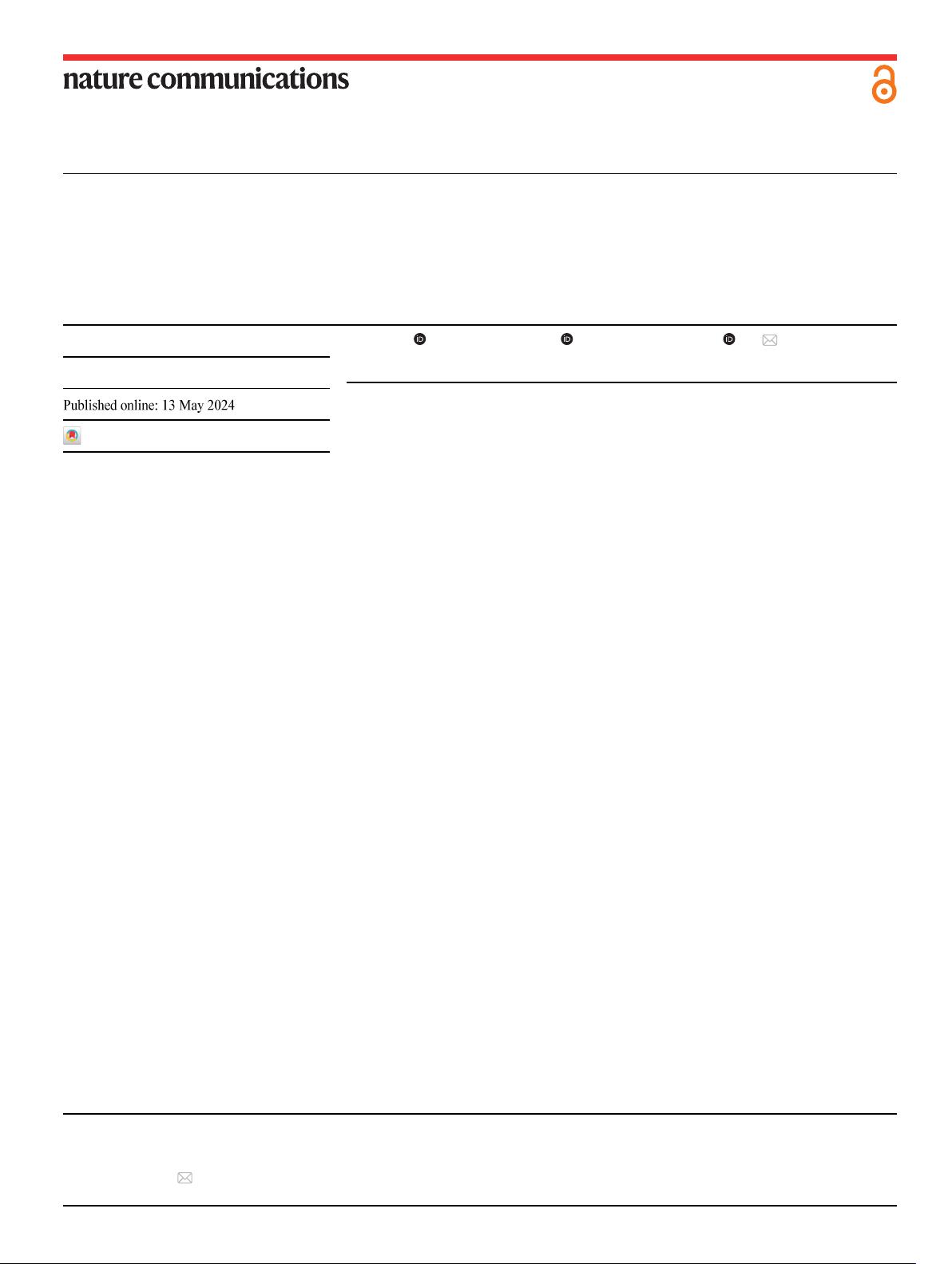
Article https://doi.org/10.1038/s41467-024-483 65-3
The structural basis for 2′−5′/3′−5′-cGAMP
synthesis by cGAS
Shuai Wu
1
,SandraB.Gabelli
1,2,3,5
& Jungsan Sohn
1,2,4
cGAS activates innate immune responses against cytosolic double-stranded
DNA. Here, by determining crystal structures of cGAS at various reaction
stages, we report a unifying catalytic mechanism. apo-cGAS assumes an array
of inactive conformations and binds NTPs nonproductively. Dimerization-
coupled double-stranded DNA-binding then affixes the active site into a rigid
lock for productive metal•substrate binding. A web-like network of pro-
tein•NTP, intra-NTP, and inter-NTP interactions ensures the stepwise synthesis
of 2′−5′/3′−5′-linked cGAMP while discriminating against noncognate NTPs and
off-pathway intermediates. One divalent metal is sufficient for productive
substrate binding, and capturing the second divalent metal is tightly coupled
to nucleotide and linkage specificities, a process which manganese is preferred
over magnesium by 100-fold. Additionally, we elucidate how mouse cGAS
achieves more stringent NTP and linkage specificities than human cGAS.
Together, our results reveal that an adaptable, yet precise lock-and-key-like
mechanism underpins cGAS catalysis.
Cyclic GMP-AMP (cGAMP) synthase (cGAS) is essential for the host
defense against cytosolic double-stranded (ds)DNA arising from var-
ious maladies (e.g., pathogen invasion, ionizing irradiation, and gen-
otoxic chemicals)
1–3
. Upon directly binding to and dimerizing on
dsDNA, cGAS cyclizes ATP and GTP into 2′−5′/3′−5′-linked cGAMP, a
unique metazoan second messenger for initiating type-I interferon
(IFN-I)-mediated inflammatory responses (Fig. 1A)
1,4–7
.cGASiscentral
to antitumor immunity, host defense against an array of pathogens,
and regulating autoimmunity
2,3,8
.
It is increasingly appreciated that cGAS-like enzymes have an
ancestral origin and cGAMPs are widely employed as second messen-
gers for both prokaryotic and eukaryotic innate immune pathways
9
.
However, only metazoan enzymes generate the 2′−5′/3′−5′-linkage
combination
4,5,9,10
, and currently, the mechanisms by which cGAS
specifically generates this uniquely linked cyclic dinucleotide remain
poorlyunderstood. Persisting questions include: how cGAS specifically
recognizes ATP and GTP at each substrate binding pocket and coor-
dinates its signature 2′−5′ linkage formation; how the same substrate
binding sites then switch their nucleotide specificity and precisely
position the GTP-AMP (pppGpA) intermediate for the second 3′−5′-
linkage formation while barring the cyclization of other off-pathway
dinucleotides; how dsDNA and dimerization regulate these processes,
how it utilizes different divalent metals; and finally, how active site
reactivity and promiscuity are regulated across different mammalian
species. Here, we resolve these numerous fundamental mechanistic
questions in innate immunology and present a unifying catalytic
mechanism of cGAS.
We find that apo-cGAS assumes an array of inactive conforma-
tions and binds ATP/GTP nonproductively without involving divalent
metals, which is then affixed into the catalytically competent con-
formation by dimerization-coupled dsDNA binding. We also delineate
how web-like network of protein•NTP, intra-NTP, and inter-NTP inter-
actions underpin the substrate-dependent linkage specificity. One
Mg
2+
is sufficient for productive substrate binding and Mn
2+
is pre-
ferred as the second catalytic metal without involving an inverted
intermediate. In the cyclization step, the adenosine of the pppGpA
intermediate binds ~30° rotated compared to the guanine of the GTP
substrate to precisely position the 3′-OH for the second linkage
Received: 31 May 2023
Accepted: 26 April 2024
Check for updates
1
Department of Biophysics and Biophysical Chemistry, Johns Hopkins University School of Medicine, Baltimore, MD, USA.
2
Department of Oncology, Johns
Hopkins University School of Medicine, Baltimore, MD, USA.
3
Department of Medicine, Johns Hopkins University School of Medicine, Baltimore, MD, USA.
4
Division of Rheumatology, Johns Hopkins University School of Medicine, Baltimore, MD, USA.
5
Present address: Discovery Chemistry, Merck Laboratories,
West Point, PA, USA.
e-mail: jsohn@jhmi.edu
Nature Communications | (2024) 15:4012 1
1234567890():,;
1234567890():,;
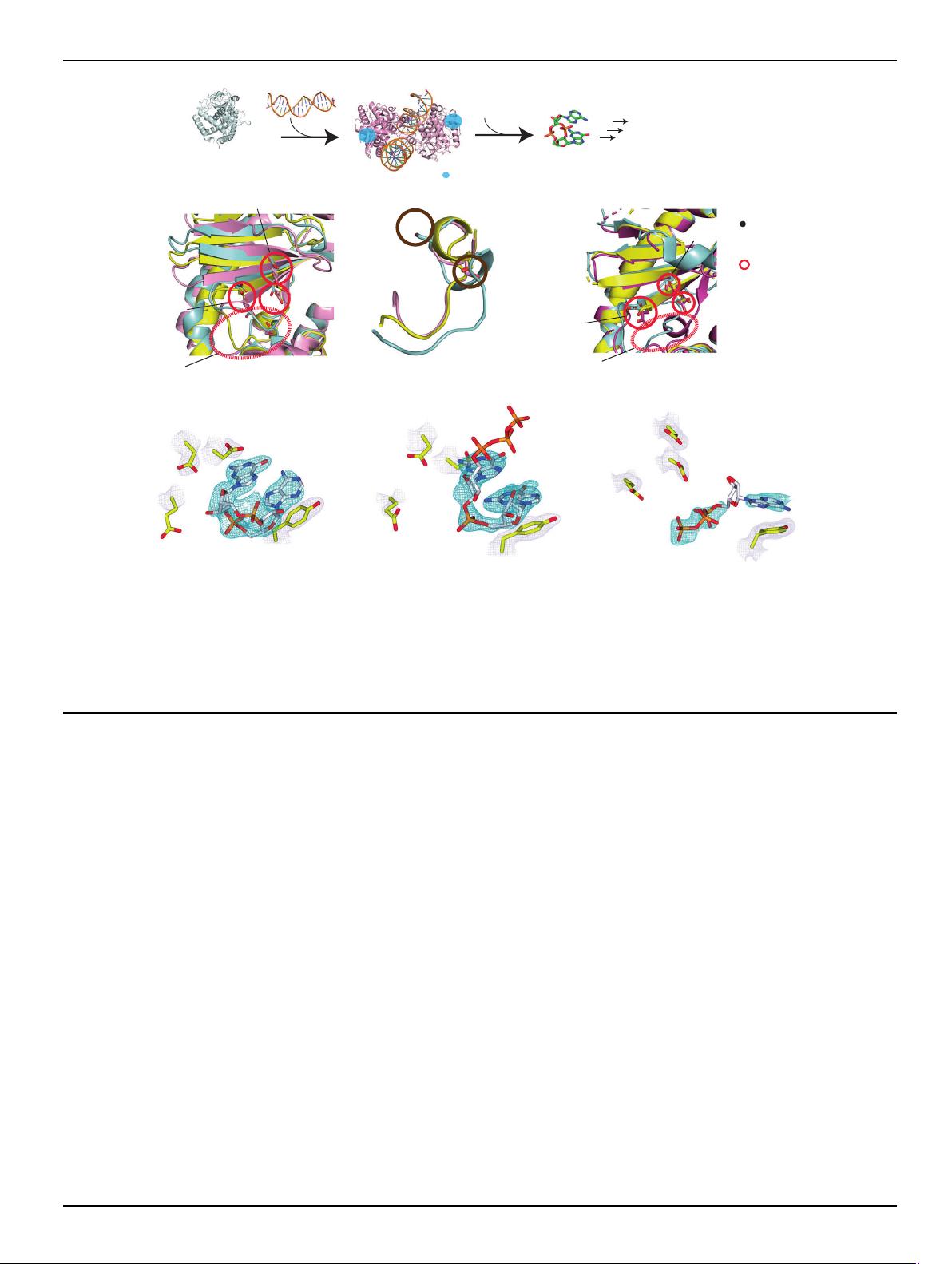
formation. cGAS employs multiple proof-reading mechanisms includ-
ing an intermediate-dep endent nucleosi de trap to prevent the cycli-
zation of nonc ognate dinucleotides. Finally, we r eveal how mouse
cGAS achieves more stringent substrate selectivity and higher catalytic
efficiency than the human enzyme. Together, we set forth a unifying
catalytic mechanism of cGAS in which dsDNA-binding pre-organizes
the active site into a rigid yet adaptable lock for two sets of keys
(substrates and intermediate) necessary to specifically synthesize 2′−5/
3′−5′-cGAMP.
Results
Activation of cGAS entails a disorder-to-order transition
Previous structural studies suggested that the active site of apo-cGAS
is occluded from binding ATP/GTP (we denote a 1:1 mixture of NTPs
and NTP/NTP hereafter). dsDNA binding reconfigures the “activation
loop” and the three β-sheets that harbor catalytic acidic residues for
coordinating NTPs and two Mg
2+
ions from the inactive to active
conformation while allowing substrate binding
4,7
. We had intended to
decipher why nucleic acids other than dsDNA bind cGAS but fail to
activate the enzyme (e.g., refs. 11,12) by crystallizing the catalytic
domain of mouse (m)cGAS
(cat)
with double-stranded (ds)RNA. How-
ever, mcGAS
cat
crystallized without the ligand, which instead provided
us with an independent view of apo-mcGAS
cat
at 1.7 Å resolution.
Here, the three β-sheets at the active site did not align particularly
well with either dsDNA-free or dsDNA-bound mcGAS
cat 4
.(Fig.1Band
Supplementary Fig. 1A), while the activation loop aligned better with
mcGAS
cat
•dsDNA (Ser
199
;Fig.1B/C). Moreover, the active site appeared
entirely solvent accessible, as the cavity volume was ~1000 Å
3
greater
than that of mcGAS
cat
•dsDNA (Supplementary Fig. 1B). To further
examine these discrepancies, we crystallized and solved the structure
of the catalytic domain of human cGAS (hcGAS
cat
). We noted that,
unlikethemouse enzyme, the three β-sheets do not move upon dsDNA
binding, while the catalytic residues assume different rotamer con-
formations (Fig. 1D and Supplementary Fig. 1A)
13,14
. However, the acti-
vation loop in our structure was mostly disordered (thus not modeled
in Fig. 1D and Supplementary Fig. 1A). Comparing the B-factors of
hcGAS
cat
structures with or without bound dsDNA revealed that, while
the C-lobe remains steady (likely via dimerization/crystal contact),
dsDNA drastically stabilizes the N-lobe containing the activation loop
and the catalytic residues (Supplementary Fig. 1C, D). Together, our
observations suggest that, instead of being fixed into a specificauto-
inhibited conformation
4,7
, the active site of resting cGAS assumes an
array of inactive states and its activation entails a dsDNA-dependent
disorder-to-order transition.
dsDNA modulates productive vs. nonproductive substrate
binding
To understand why dsDNA-free cGAS is only basally active despite the
open active site
15
, we attempted to capture substrate-bound cGAS
without dsDNA. After conducting co-crystallization and soaking trials,
cytosolic dsDNA
Asp
307
Asp
213
Glu
211
•
•
•
Ser
199
Ser
Ser
199
199
Ser
Ser
199
199
apo
apo
-mcGAS
-mcGAS
cat
cat
(
8shu)
8shu)
vs. apo
apo
-mcGAS
-mcGAS
cat
cat
(4k8v)
(4k8v)
/mcGAS
mcGAS
cat
cat
•dsDNA (4k96)
•dsDNA (4k96)
Asp
227
Glu
225
Asp
319
B
C
D
Asp
307
Glu
211
Asp
227
Glu
225
Asp
319
Tyr
436
cGAMP
hcGAS
cat
E
F
G
Asp
227
Glu
225
Asp
319
Tyr
436
pppGpG
hcGAS
cat
Asp
213
mcGAS
cat
Tyr
421
ATP
apo
apo
-hcGAS
-hcGAS
cat
cat
(4km5)
(4km5)
hcGAS
hcGAS
cat
cat
•dsDNA (6ct9)
•dsDNA (6ct9)
•
•
•
apo
apo
-hcGAS
-hcGAS
cat
cat
(8shz)
(8shz)
Ser
Ser
199
199
inammatory
responses
cGAS
(catalytic domain)
cGAMP
ATP/GTP
A
“activation loop”
“activation loop”
+(Mg
2+
•ATP/GTP) (8si0)
+(Mg
2+
•GTP/GTP) (8sj8)
+(Mg
2+
•ATP/ATP) (8shk)
(this work)
(this work)
= active site
= position of 3
E-sheets
= catalytic
residues
Fig. 1 | The activation of cGAS entails a disorder-to-order transition. A Cartoon
describing the activation of cGAS. B, C An overlay of dsDNA-free and dsDNA-bound
wild-type (WT)-mcGAS
cat
structures at the active site (B) and the activation loop (C).
PDB ID: 8shu (yellow) is from this work. The PDB ID for each structure is appended
in parenthesis hereafter and Supplementary Tables 1–9 list all data collection and
refinement statistics. D An overlay of dsDNA-free and dsDNA-bound WT-hcGAS
cat
structures. The “activation loop” in the new structure from this work (PDB ID: 8shz,
yellow) is not modeled due to poor electron density. E dsDNA-free WT-hcGAS
cat
bound to cGAMP. The 2Fo-Fc map is contoured at 1.5 σ (same for all 2Fo-Fc maps
shown hereafter). The Fo-Fc (omit)maps for all NTPs are shown in Fig. S8. F dsDNA-
free WT-hcGAS
cat
bound to pppGpG. The triphosphate was modeled in the absence
of electron densities for presentation. G dsDNA-free WT-mcGAS
cat
bound to ATP.
The ribose was modeled without density for presentation.
Article https://doi.org/10.1038/s41467-024-48365-3
Nature Communications | (2024) 15:4012 2
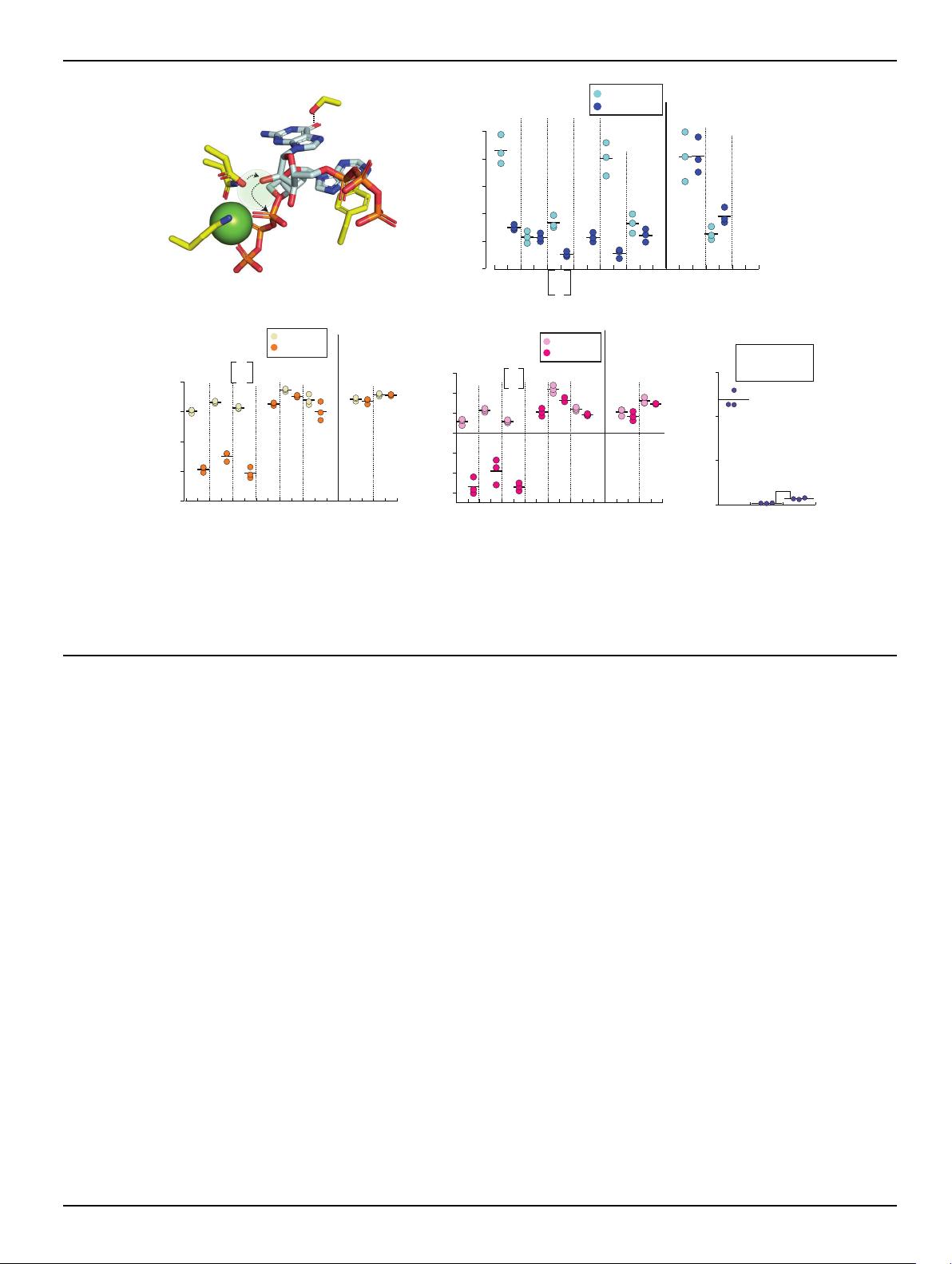
we obtained the following structures with either human or mouse
cGAS
cat
. We co-crystallized hcGAS
cat
with Mg
2+
+ ATP/GTP without
dsDNA and found a new density corresponding to cGAMP at the active
site; the catalytic residues remained in the inactive state (Fig. 1E; 5 mM
Mg
2+
is present in all our soaking/biochemical experiments unless
noted otherwise). Co-crystallizing hcGAS
cat
with GTP resulted in the
pppGpG dinucleotide (GTP-GMP) bound in a conformation unamen-
able to further reaction: the densities for the triphosphate and Mg
2+
were missing and the catalytic residues remained in the inactive state
(Fig. 1F). Also of note, the active site B-factors remain high despite
bound NTPs in both structures (Supplementary Fig. 1D). We next
solved the crystal structure of ATP-bound mcGAS
cat
without dsDNA via
soaking. Here, one ATP was clearly bound at its designated binding
pocket (Site-1), but the second NTP binding site (Site-2) remained
empty (i.e., the GTP binding site; Fig. 1G). Strikingly, electron densities
were missing for both the ribose of ATP and Mg
2+
; the catalytic residues
stayed in the inactive state and the triphosphate appeared suboptimal
for coordinating Mg
2+
(Fig. 1G and Supplementary Fig. 1E). These
results are consistent with the mechanism in which cGAS binds NTPs
without dsDNA
15
, but it is only basally active because it can rarely fix
NTPs and metals into the catalytically competent conformation (i.e.,
nonproductive binding).
dsDNA binding provides enthalpy for productive substrate
binding
Our observation that dsDNA-free cGAS binds NTPs nonproductively
prompted us to reinvestigate the role of dsDNA in catalysis. We largely
employ mcGAS
cat
hereafter as its dsDNA-bound form was conducive to
crystallization and binding assays using isothermal titration calori-
metry (ITC). To generate a cGAS construct that binds NTPs but is
deficien t in catalysis, we neutralized two acidic residues that coordi-
nate Mg
2+
•ATP
16
(E211Q-D213N in mcGAS, denoted as QN hereafter),
which essentially abrogated the enzymatic activity (Supplementary
Table 10). Soaking QN-mcGAS
cat
•dsDNA crystals with ATP/GTP resul-
ted in one Mg
2+
and each NTP bound in position for the first 2′−5′-
linkage formation (Fig. 2A and Supplementary Fig. 2A; the loss of the
second Mg
2+
was likely caused by the mutation, thus precluding cata-
lysis). Soaking QN-mcGAS
cat
•dsDNA crystals with GTP showed two
GTPs in the catalytically competent state (Supplementary Fig. 2B).
Moreover, soaking ATP to QN-mcGAS
cat
crystallized with and without
dsDNA showed the productive (one Mg
2+
)andnonproductive(no
Mg
2+
) binding states seen from WT ± dsDNA (two Mg
2+
and no Mg
2+
),
respectively (Fig. 1G and Supplementary Fig. 2C–E). These observa-
tions corroborate thatthe QN mutant is well-suitedfor delineatinghow
dsDNA regulates substrate binding.
Consistent with our crystal structures (Fig. 1E–G), QN-mcGAS
cat
bound NTPs in µMaffinities (K
D
s) in the absence of dsDNA (Fig. 2B;
values are listed in Supplementary Table 11 and representative ITC
traces are shown in Supplementary Fig. 7). When QN-mcGAS
cat
was pre-
complexed with dsDNA, not only did the K
D
improve (Fig. 2B), but the
overall thermodynamic profile also changed drastically (Fig. 2B–D).
For example, when bound to dsDNA, the ΔH of ATP binding decreased
by ~10 kcal/mol and the ΔS decreased by 30 cal/mol/deg. The favorable
ΔΔH caused by dsDNA binding corroborates the formation of new
interactions between the repositioned catalytic residues and
Mg
2+
•ATP, and the unfavorable ΔΔS supports the disorder-to-order
transition observed in crystal structures (e.g., Supplementary Fig. 2C
vs. 2D; e.g., ref. 17). Also of note, not only was cGAMP binding barely
affected by dsDNA, but it also bound more weakly than ATP/GTP,
suggesting that the product would not interfere with catalysis
-30
-20
-10
0
10
20
30
0
10
20
30
40
50
Asp
307
Gln
211
Asn
213
Ser
366
Tyr
421
ATP
Site-1
Site-2
GTP
A
C
ΔH (kcal/mol)
ΔS (cal/mol/deg)
K
D
(μM)
ATP
GTP
(GTP)
ATP
(ATP)
GTP
cGAMP
ATP
GTP
ATP
GTP
ATP GT P
ATP
(GTP)
GTP
(ATP)
cGAMP
ATP
GTP
(-) dsDNA
(+) dsDNA
ATP GTP
ATP
(GTP)
GTP
(ATP)
cGAMP
ATP
GTP
ATP
GTP
(-) dsDNA
(+) dsDNA
N.B.
N.B.
QN-mcGAS
cat
K382E/
QN-mcGAS
cat
QN-mcGAS
cat
K382E/
QN-mcGAS
cat
QN-mcGAS
cat
K382E/
QN-mcGAS
cat
N.B.
N.B.
ATP
GTP
(-) dsDNA
(+) dsDNA
(GTP) + ATP
(ATP) + GTP
N.B.
N.B.
N.B.
N.B.
D
E
NTase rate (enz
-1
min
-1
)
ATP/GTP
ATP/ATP
GTP/GTP
WT-mcGAS
cat
•dsDNA
B
N.B.
N.B.
QN-mcGAS
cat
•dsDNA (7uxw)
0
2
4
6
-20
-15
-10
-5
0
***
Fig. 2 | dsDNA regulates productive vs. nonproductive substrate binding. A The
active site of E211Q/D213N (QN)-mcGAS
cat
•dsDNA in complex with ATP and GTP.
The position for the missing second Mg
2+
was shown as a shaded circle. Dotted
arrows indicate the deprotonationand subsequent nucleophilic attack by the 2′-OH
of GTP for the first linkage formation. The H-bond between Ser
366
and the carboxyl
of GTP is also indicated. B The binding affinity (K
D
) of mcGAS
cat
toward various
NTPs with orwithout dsDNA (18-bp for all) was determined byITC. The NTPs in gray
parentheses are precomplexed with cGAS ± dsDNA. C The ΔH of mcGAS
cat
binding
various NTPs with or without dsDNA. D The ΔSofmcGAS
cat
binding various NTPs
with or without dsDNA (T = 25 °C). E The catalytic activity of WT-mcGAS
cat
•dsDNA
(60-bp dsDNA) toward various NTPs. **p =0.007.Allp values hereafter are deter-
mined by Student’s t test using Microsoft Excel (two-tailed with equal variances).
*p < 0.05; **p < 0.01; ***p <0.001.
Article https://doi.org/10.1038/s41467-024-48365-3
Nature Communications | (2024) 15:4012 3
剩余12页未读,继续阅读
资源推荐
资源评论
资源评论
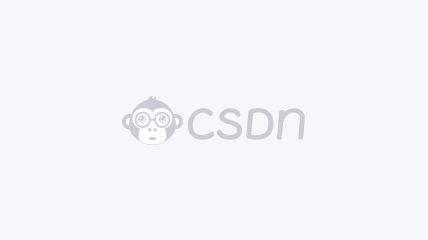

m0_59100648
- 粉丝: 0
- 资源: 1
上传资源 快速赚钱
我的内容管理 展开
我的资源 快来上传第一个资源
我的收益
登录查看自己的收益我的积分 登录查看自己的积分
我的C币 登录后查看C币余额
我的收藏
我的下载
下载帮助

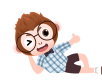
最新资源
- 基于Comsol三次谐波的物理现象,大子刊NC复现报告:手性BIC超表面下的远场偏振与手性透射图示分析-电场、二维能带图解读及Q因子图展现所见即所得的光学效应 ,平面手征超表面研究:连续介质中的三次
- 人工智能&深度学习:LSTM 文本分类实战 - 基于 THUCNews 数据集的 Python 源码资源(源码+数据集+说明)
- MATLAB程序专为非全向移动机器人设计的扩展卡尔曼滤波(EKF)数据处理工具箱,精准融合ADS-B与GPS数据,高效状态估计解决方案,MATLAB程序优化:非全向移动机器人EKF状态估计与飞行数据处
- 简易图像处理软件,与PS工具类似
- iOS swift工具类使用
- AR.js 完整资源包,可以完整的引用
- 西门子PLC与三台欧姆龙温控器通讯程序:实现温度控制及监控,支持轮询通讯与故障恢复功能,PLC与触摸屏集成设置温度,支持扩展及详细注释 ,西门子PLC与三台欧姆龙温控器通讯程序:实现温度控制及监控,支
- 这份文档的内容并非技术性文章,而是一段歌词片段,无法按照技术文档的要求生成标准标题 若需要总结,该文档包含了一段歌词,表达了关于期待与未知相遇的主题 但由于内容不足以及非技术性质,无法提供更详细总
- .safetensors转换成.GGUF所需工具cmake
- 三相光伏并网逆变器仿真:PV升压逆变并网系统中的电压电流双环控制与SVPWM策略研究,三相光伏并网逆变器仿真研究:PV光伏boost升压逆变并网系统之电压外环与电流内环SVPWM控制机制探讨,三相光伏
- 《基于信捷PLC的7轴伺服插补联动设备的设计与实现-喷涂机程序与牵引示教功能》,信捷PLC驱动7轴伺服插补联动设备-XD5-48T6-E牵引示教功能与喷涂机程序解析,信捷PLC7轴伺服插补联动XD
- MPC模型预测控制:从原理到代码实现,涵盖双积分、倒立摆、车辆运动学与动力学跟踪控制系统的详细文档与编程实践,MPC模型预测控制原理到代码实现:双积分、倒立摆、车辆运动学与动力学跟踪控制案例详解,mp
- 车路协同C-V2X港口应用分析
- gradle-6.1.1.zip资源下载
- 用dockerfile打包带有nginx-monitor-vts模块的nginx镜像
- .safetensors转换成.GGUF所需工具ccache
安全验证
文档复制为VIP权益,开通VIP直接复制
