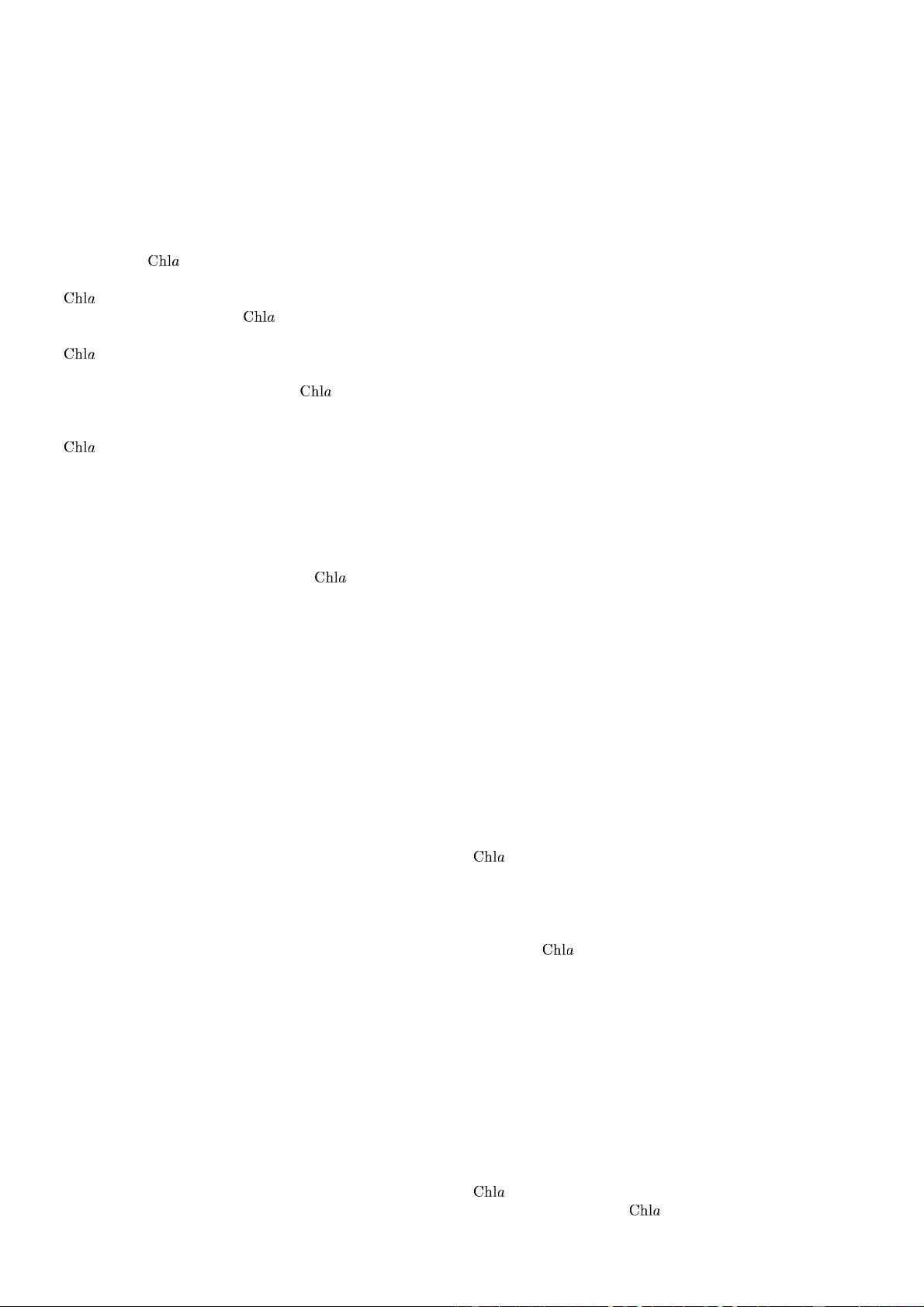
IEEE Proof
Web Versi
2 IEEE JOURNAL OF SELECTED TOPICS IN APPLIED EARTH OBSERVATIONS AND REMOTE SENSING
scale [11]. Thus, the coastal w aters are referred to as Case II
waters. In these waters, several constituents of different origins
and optical b ehaviors have an influence on the water color
and vary more or less independently, i.e., the inherent optical
properties are determined not only by phytoplankton, but also
absorption and scattering by TSM and CDOM, which do not
covary with
.[9].Asaresult,thesamewater-leaving
reflectance in turbid waters is likely to correspond to different
concentration, because the optical properties of waters
are not only dom inated by
and cov arying detrital pig-
ments, but depend on other substances that don’t covary with
. S uch substances include CDOM, suspended sediments,
coccolithophores, detritus, and bacteria [12]. Consequently, it
is q uite difficult to accurately retrieve
concentration from
turbid coastal waters, and present water color remote sensing
researches focus on how to improve the estimation accuracy of
concentration in coastal waters.
In general, the w ater-leaving reflectance detected by satellite
sensor is fairly weak. For example, the component of the mea-
sured reflectance, backscattering out of the w ater and transmit-
ting to th e top of the atmosphere, is less than 1 5% in the blue
band, particularly much smaller in the green and near-infrared
ranges [13]. Moore et al. [14] pointed out that approximately
35% of uncertainty could be generated in
concentration es-
timation while the atmospheric correction uncertainty could be
up to 5%. Moreover, as part of future oceanic plans, retrieval of
water-leaving reflectance with 5% uncertainty is recommended
to be one of the m ain m ission s of National Aeronautics and
Space Administration (N ASA) [15]. The NASA’s mission has
been achieved in Case I w aters, but may fail in the Case II wa-
ters, because turbid water constituents (TSM, bubbles, etc, or
bottom reflectance) can contribute significant amounts of radi-
ance to the atmospheric correction bands. [16]–[18]. This will
induce large errors w hen the standard atmospheric correction
scheme is adopted, since the water-leaving radiance is assumed
to be negligible in n ear-infrared part of the spectrum [19]. Given
these factors, it is easy to understand why much attention has
been paid to the atmospheric correction.
Scale is not something new, nor is involved just by geog-
raphy, cartography or geographic information science. Scale
refers generally to the detailing proportion, by which informa-
tion can be observed, represented, analyzed and communicated
[20]. However, this old concept undergoes some fundam ental
new changes as a result of remote sensing techno log y. The
scale represents th e window of perception, the ab ility of ob-
servation, and the limitation on the perception or view of a
phenomenon [21]. Consequently, issu es regarding the scale
should be carefully dealt with in remote sensing. On one hand,
most retrieval models and algorithm s are basically derived at
small scales, implying that the water surface is homogeneous. If
large scales are utilized, certain uncertainty may arise [22]. O n
the other hand, the ecological process occurring in the aquatic
environment m ust be described at a certain scale, implying that
small scale information cannot be sim ply used as a substitute
for local scale inform ation. As a result, the scaling of geo-infor-
mation is inevitable to observe many disciplines. Scale effects
constrain the accuracy of retrieval and limit the development of
remote sensing applications [21]. Consequently, they become
the emerging problem of scientists’ concern in o cean color
remote sensing.
II. A R
EVIEW ON OCEAN COLOR SENSORS FOR
COASTAL WATER S
The prelim inary stage of ocean color sensors’ development
lasts from the late 1950s to early 1970s. It began in 1957 when
the first artificial satellite, Sputnik, was launched by the Soviet
Union. Sputnik marked inn ovation and expansion in Earth ob-
servation as well as space exploration. In 1960, the first Soviet
Union meteorological satellite was in orbit, and then a historic
conference was held in 1964 at Woods Hole Oceanographic In-
stitute, to discuss the po ssible ways in which Earth-observation
satellites might contribute to the science of oceanography [23].
Indeed it is surprising that the tremendous development of ocean
remote sensing in the last quarter of the 2 0th century was already
envisaged by a previous generatio n of oceanograp hers. Right
from the beginning it was recognized that the vantage point of
Earth orbit offered new spatial view of the ocean, and would re-
lease oceanograph ers from the sampling lim itation s of ship s and
buoys, since they had been restricted to considering only “prob-
lems that we re derived from the vertical distribution of proper-
ties at stations widely separated in space and time” [24]. During
the late 1960s and 1970s, exp erimental Earth-observing satel-
lites provided technological experience, and the meteorological
satellites began to play a part in operational forecasting [23]. In
this stage, m ost of the observations from space came in pho-
tographic form or televisio n picture, and the d igital image pro-
cessing technology was gradually applied in the remote sensin g
to analyze the largely qualitative information measured in space.
The period from the late 1970s to early 19 90s is a rapid
development stage. In 1978, two satellites were launched by
NASA with the aim not only to prove the concepts proposed
in the 1960s, b ut also to deliver quan titativ e, calibrated mea-
surements of the ocean. Two sensors, N ational Oceanic and
Atmospheric Administration (NOAA) sensor and Advanced
Very High Resolution Radiometer (AVHRR), were boarded
on th ese satellites. Clark et al. [25] demonstrated that the
concentration in the surface waters of the ocean could
be deduced from the spectrum of upw elling light from the
ocean, the “ocean color”. Subsequently, NASA launched the
Coastal Zone Color Scanner (CZCS) on Nimbus-7 in late 1978.
The CZCS w as a proof-of-concept mission with the goal of
measuring
concentration from space [13]. Unfortunately,
just as ocean scientists began to realize in the 1980s that
sensors in space were not sim ply interesting novelties but
essential for the development of their research on a g lobal
scale, the generous funding for space technology had gone
down th e drain [23]. In the late 1980s, new organ izations
and institutions joined ocean observation plans. For example,
both Europe and Japan inaugurated series of Earth-observing
satellites, which have been running until now, and yielded d ata
of rich value to oceanic researchers. In this stage, measurement
specifications such as “ocean optical protocols for satellite
color sensor validation” were established and implemented.
The “clear water” atmospheric correction algorith m and global
concentration estimation al gorithm s were constructed and
developed for retrieving
concentration on a global scale.